Advancing Aerospace and Defense Innovation: TA Instruments’ Material Characterization Portfolio
Sam Barnes | Abhinandh Sankar
May 16, 2025
Innovation in materials science is a continuous pursuit, driven by the demand for lighter, stronger, more durable, and more reliable components. This is especially critical in the aerospace and defense industries, where materials must perform reliably under extreme conditions—including intense mechanical stress, wide temperature fluctuations, and long-term environmental exposure.
But how do scientists and engineers ensure these materials will perform as intended? The answer lies in precise material characterization.
TA Instruments | Waters offers a comprehensive suite of thermal analysis, rheology, and mechanical testing instruments designed to meet the rigorous demands of military and aerospace applications. Whether you are validating adhesive cure profiles using rheological tools like the Discovery Hybrid Rheometer (DHR) or using thermal analyzers such as the DSC 2500 for optimizing composite cure cycles, our portfolio enables end-to-end material qualification. Complementary techniques such as advanced dilatometry (DIL), dynamic mechanical analysis (DMA), and microcalorimetry (TAM) further support material development, optimization, and certification under extreme operational conditions.
Together, these tools form a powerful, integrated portfolio that supports every stage of materials innovation—from early research and development to final performance validation. By enabling deeper insights into material behavior under real-world conditions, TA Instruments empowers aerospace and defense organizations to push the boundaries of performance, ensure mission-critical reliability, and accelerate innovation across the entire value chain.
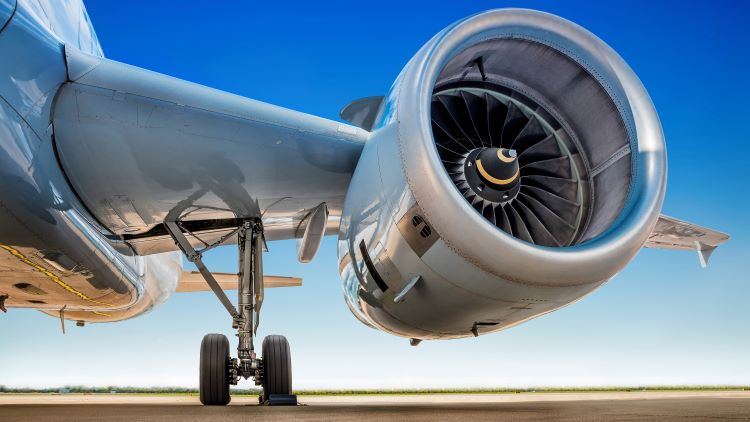
How Advanced Material Characterization Drives Aerospace and Defense Performance
Aerospace and defense materials must withstand incredible stresses, extreme temperatures, and demanding environments while minimizing weight and maximizing reliability. Here’s how a synergistic approach to material characterization—combining rheology and thermal analysis—supports every stage of an aerospace component’s lifecycle.
1. Innovating the Future: Advanced Materials Research & Development
The journey to next-generation aerospace materials begins with deep insight into their fundamental properties.
Rheology: Analyzing Flow and Form
Before a material becomes a high-performance component, understanding its flow behavior in fluid or semi-solid states is essential.
- For polymers and composite resins, rheometers measure key properties such as viscosity under varying temperatures and shear rates, viscoelastic response to stress, and behavior throughout the curing process. This information is crucial for designing resins that flow predictably into complex molds, ensuring complete fiber wet-out and void-free composite structures.This becomes especially important with B-stage or prepreg resins—materials that are partially cured to allow for easier handling, layup, and storage before final curing. Rheological testing helps manufacturers fine-tune the degree of partial cure to balance tackiness for ply placement with enough rigidity for dimensional stability. Understanding how these resins behave under thermal and mechanical stress ensures they process correctly during layup, autoclaving, or out-of-autoclave consolidation, leading to strong, reliable aerospace-grade composites.
- When developing specialized coatings or advanced slurries, rheology guides formulators in achieving stable dispersions and application characteristics such as sprayability, film uniformity, and leveling. This is especially critical in aerospace and defense applications, where coatings often serve functional, not just protective, roles. Examples include:
- Radar-absorbing materials (RAM) used on stealth aircraft or naval vessels to reduce radar cross-section.
- Thermal barrier coatings applied to turbine engine components to withstand extreme heat and oxidative environments.
- Anti-corrosive and anti-icing coatings for aircraft skins, rotor blades, or ship surfaces to improve durability and operational readiness.
- Ablative coatings for missile or re-entry vehicle surfaces that slowly erode to absorb intense heat during high-speed atmospheric transit.
Rheological data ensures these formulations maintain the right consistency during application, adhere properly, and cure uniformly—ultimately determining their real-world effectiveness and longevity.
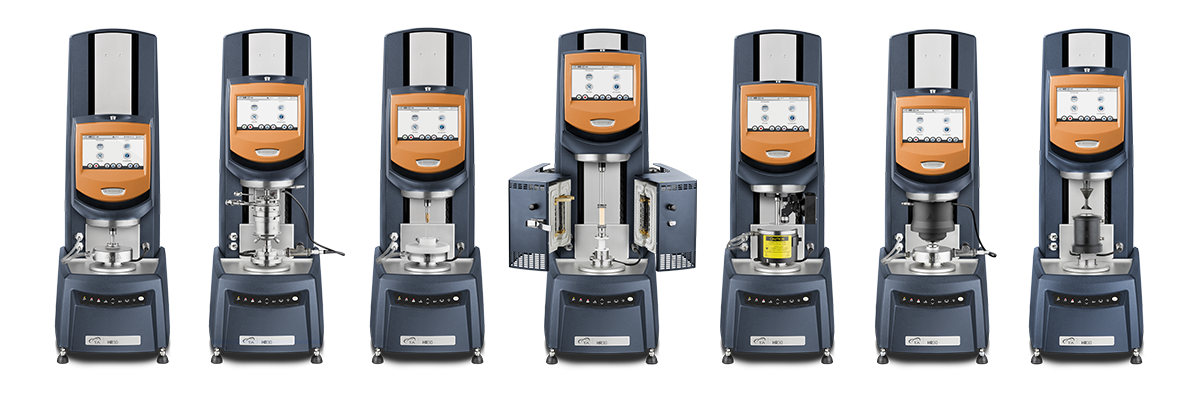
DSC & TGA: Defining Thermal Fingerprints
As materials take shape, their thermal characteristics reveal how structure relates to performance. Thermal analysis exposes materials to application-relevant temperatures and then measures how heat flows through the material and changes its properties, such as weight change. Thermal analysis complements rheological data by uncovering transitions such as melting, crystallization, and degradation – key factors in ensuring optimal performance in demanding aerospace and defense environments
- Differential Scanning Calorimetry (DSC) pinpoints critical thermal transitions—including glass transition temperature (Tg), melting points (Tm), and heats of transition. This data shapes processing windows and defines safe operational limits.
- Thermogravimetric Analysis (TGA) complements DSC by tracking mass changes with temperature. It identifies decomposition onset, quantifies critical components (like resin vs. fibers), and detects volatiles or moisture—crucial insights for composites destined for high-temperature or high-performance environments.
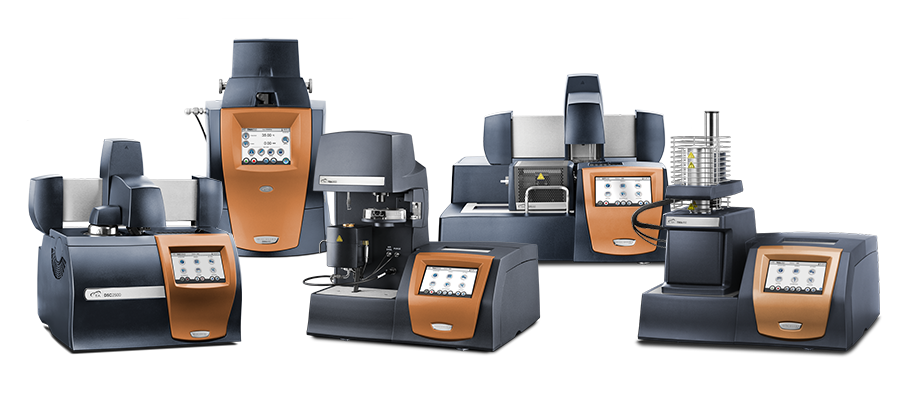
Measuring Thermal Conductivity
A material’s ability to manage heat is often a primary design driver in demanding aerospace and defense conditions. Our instruments measure thermal conductivity to help engineers select or develop materials that dissipate heat from sensitive electronics or insulate critical structures from extreme temperatures.
Dilatometry: Predicting Thermal Expansion
Materials expand and contract with temperature. Dilatometry precisely measures thermal expansion—vital when joining dissimilar materials, a common scenario in aerospace. Mismatched coefficients of thermal expansion (CTE) can induce stress and compromise structural integrity.
2. Building with Precision: Manufacturing & Process Optimization
Translating innovative materials into reliable, high-volume production demands meticulous process control, informed by detailed material characterization.
Optimizing Processability with Rheology
For composite manufacturing methods like resin transfer molding or automated fiber placement, rheology is key to understanding how resins flow, impregnate fibers, and cure over time and temperature—ensuring void-free, structurally sound parts.
Monitoring Cure and Thermal History with DSC & DMA
- For thermosetting materials, achieving the correct degree of cure is critical. DSC monitors residual cure energy, enabling optimized cure cycles for quality and efficiency.
- DMA tracks mechanical property development—like stiffness and damping—throughout the cure process and across temperatures, offering added confidence in material performance.
Both rheological profiles (viscosity, modulus) and thermal signatures (DSC curves, TGA weight loss profiles) serve as “fingerprints” for raw materials. These fingerprints are crucial for quality control because they allow manufacturers to verify that each batch of material meets strict performance specifications. By comparing these profiles to established benchmarks, any deviation can be quickly identified, helping to prevent defects, ensure consistency, and maintain compliance with industry standards.
3. Ensuring Operational Performance & Long-Term Reliability
Once deployed, aerospace components must perform flawlessly—often for decades. Characterization prior to use is essential to understand in-service behavior and predict lifespan.
Assessing In-Service Stability with TGA & DSC
- TGA and DSC assess thermal and oxidative stability, helping engineers track how engine components, thermal protection systems, or polymeric housings endure real-world thermal stresses and atmospheric exposure—identifying early signs of degradation.
Simulating Dynamic Aerospace Environments with ElectroForce APEX-1
Aerospace components face dynamic, repetitive stresses. The ElectroForce APEX-1 system delivers critical insights by simulating both small, repeated stresses and larger, infrequent events.
- This system enables high-resolution displacement control, supporting studies of fracture mechanics and fatigue propagation.
- Data from APEX-1 refines fatigue models and enhances lifecycle predictions for current and future aerospace designs.
Detecting Subtle Degradation with Isothermal Microcalorimetry (TAM)
For safety, shelf-life prediction, and hazard assessment—especially in munitions and energetic materials—Isothermal Microcalorimetry (TAM series) provides unmatched sensitivity.
- It detects early decomposition or unwanted chemical activity in materials during long-term storage or deployment.
- This is crucial for surveillance testing and quality control per standards like STANAG 4582.
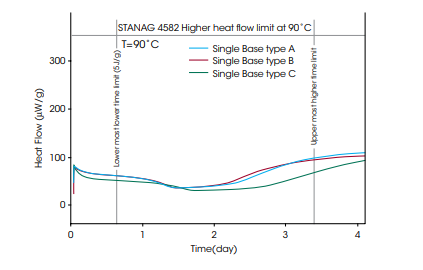
Conclusion
By integrating insights from rheology, comprehensive thermal analysis, and advanced dynamic mechanical testing—including instruments like the ElectroForce APEX-1—the aerospace industry can achieve a profound understanding of material performance from initial design through decades of service.
Frequently Asked Questions (FAQ)
What materials testing methods are used to evaluate composites in aerospace applications?
Composites used in aerospace are evaluated using a combination of rheology, dynamic mechanical analysis (DMA), thermal analysis, and thermomechanical analysis (TMA). These methods help measure properties such as stiffness, curing behavior, thermal stability, and expansion under temperature changes. For example, DMA is commonly used to assess how aerospace composites maintain structural integrity across a wide temperature range, while rheometers characterize the flow behavior of resins during processing.
How do you test the thermal stability of military-grade polymers and adhesives?
To assess thermal stability, materials scientists use instruments like Thermogravimetric Analysis (TGA) and Differential Scanning Calorimetry (DSC). TGA measures weight loss as a material is heated, identifying decomposition temperatures and compositional changes. DSC detects heat flow associated with melting, glass transitions, and curing. These techniques are critical for validating adhesives and polymers used in missiles, airframes, and field-deployable electronics.
What role does rheology play in defense material development?
Rheology is vital for understanding how materials behave under stress, which is essential for developing coatings, lubricants, sealants, and additive manufacturing feedstocks used in defense applications. Rheometers help engineers optimize formulations for extreme environments, such as high-altitude aircraft or desert-deployed vehicles, by measuring viscosity, yield stress, and flow stability.
How are energetic materials like explosives and propellants tested for safety?
Energetic materials are tested using techniques like isothermal microcalorimetry (e.g., TAM IV) and DSC to monitor thermal stability and detect potential runaway reactions. These tests can reveal low-level degradation over time, help estimate shelf life, and identify safe storage conditions for explosives, propellants, and solid fuels used in missiles and launch systems.
How do you evaluate the effects of extreme environments on aerospace materials?
Materials destined for extreme environments—such as space, arctic regions, or subsea operations—are evaluated with DMA, TMA, and high-pressure TGA/DSC systems. These tools simulate thermal cycling, pressure changes, and mechanical stress over time to predict fatigue, creep, and failure modes. This data supports design validation for components like satellite housings, radar domes, or submarine insulation.
What testing is required for flame-retardant materials in military applications?
Flame-retardant materials are assessed through flammability testing, smoke density analysis, and off-gassing characterization. These evaluations are essential for materials used in aircraft interiors, armored vehicle cabins, and personal protective equipment. Testing helps ensure compliance with military standards for ignition resistance, toxicity, and fire containment in confined environments.
How do you detect degradation in long-term deployed military materials?
Long-term degradation is often slow and subtle, making isothermal microcalorimetry (e.g., TAM IV) especially valuable. It detects minute heat flows caused by chemical or physical changes in composites, pharmaceuticals, or energy storage materials. Combined with TGA and DMA, it allows researchers to model aging processes and predict service lifetimes under various deployment scenarios.
Other Resources
- Blog – What is Dynamic Mechanical Analysis?
- Blog – What are Rheometry and Rheology?
- Blog – Linear Viscoelastic Region: Why It’s Crucial in Materials Testing
- Application Note – Measurement of Glass Transition Temperatures by Dynamic Mechanical Analysis and Rheology
- Application Note – Temperature and Frequency Trends of the Linear Viscoelastic Region
- Application Note – Introduction to Dynamic Mechanical Analysis and its Application to Testing of Polymer Solids