Keywords: Hydrogels, rheology, gelation, viscoelasticity, gel point, foods, cosmetics, biomaterials
RH124
Abstract
Hydrogel materials are widely used in the food, cosmetic, pharmaceutical and biomedical industries. They usually have high water content. The gel formation mechanism, which is also called the gelation mechanism, can be either a chemical crosslinking or a physical network association. A rheometer is a powerful instrument that can be used to monitor the gel formation and also to measure the gel strength. Two case studies are discussed regarding the use of a TA Instruments’ rotational rheometer to analyze the properties of different types of hydrogels.
Introduction
Hydrogels are generally made of natural or synthetic polymers with water. The water content of a hydrogel material can be as high as 99%. In the pharmaceutical and biomedical industries, hydrogels are mainly used as drug delivery carriers, tissue scaffolds, wound healing patches, [1-5] etc. Hydrogels are also commonly seen in our daily foods and cosmetic products [6-7]. The formation of a hydrogel can be either through chemical crosslinking or physical polymer chain association. Depending on the gelation mechanism, a hydrogel can have different mechanical and thermal properties. In order to obtain a hydrogel product that has consistent properties for a specific application, it is important to understand the chemistry of gelation and to be able to quantitatively analyze the physical properties of the gel. In this paper, a TA Instruments rotational rheometer was used to study the properties of hydrogel materials.
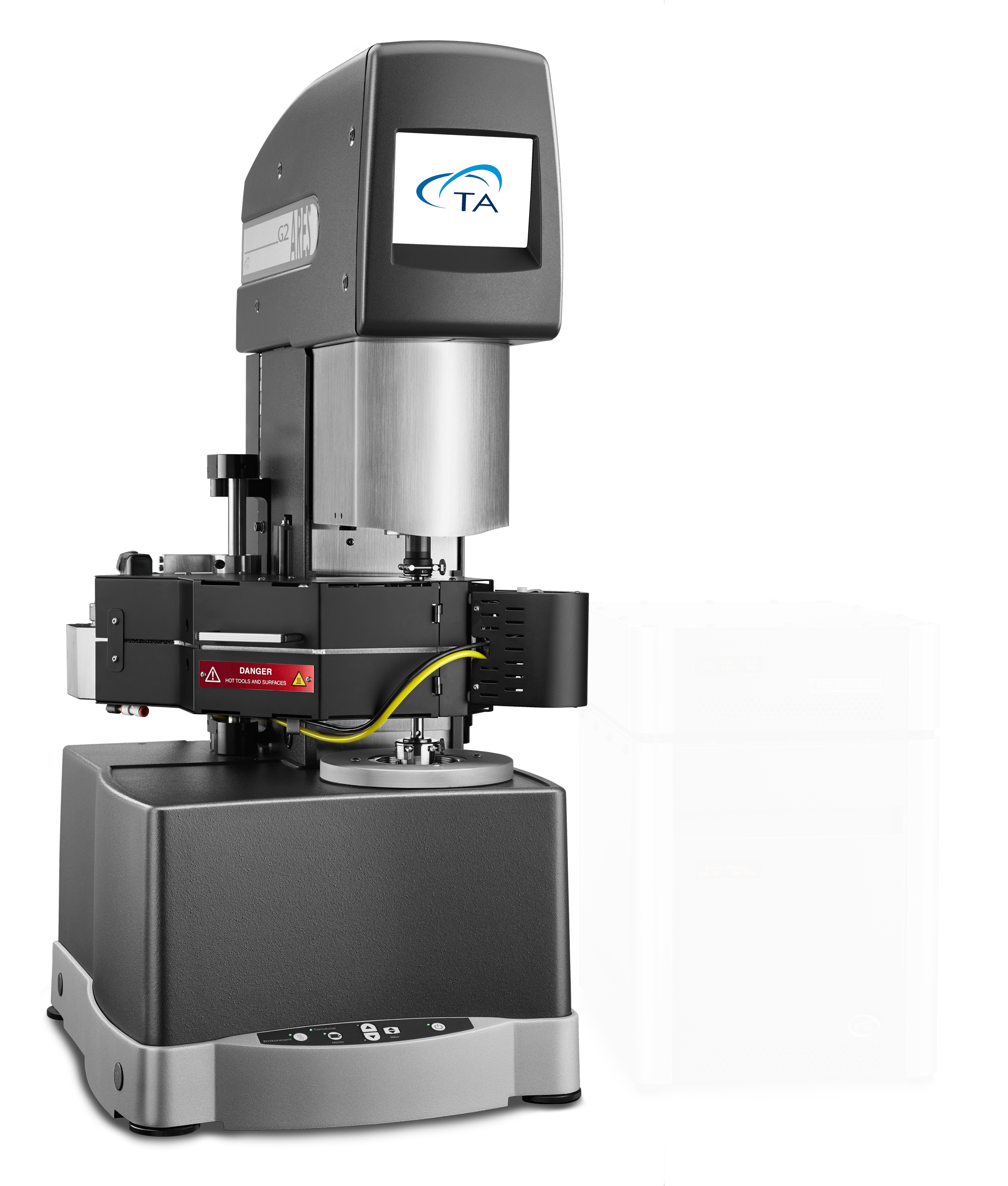
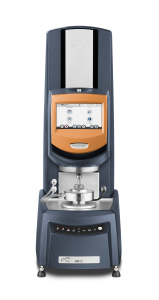
Experimental
Three hydrogels were studied in this review: a gelatin based gel, a silicon based gel, and two types of contact lenses. Gelatin (type A from porcine skin, 175 bloom) was purchased from Sigma Chemicals. A silicon based monomer solution and a crosslinking agent were provided by a bio-medical company. Contact lenses were obtained from 2 different contact lens companies.
Gelatin Based Gel
A rotational rheometer with a Peltier temperature control system was used for analyzing the viscoelastic properties of hydrogels. To prevent slip, hydrogels should be tested with a roughened plate, such as a sand blasted or crosshatched plate, shown in Figure 1. This work utilized a crosshatched Peltier plate. For monitoring the gel formation of a thermally reversible gelatin-based gel, dynamic temperature ramp down and up tests were performed at rates of 1 °C/min and 2 °C/min, respectively. A gelatin solution was loaded onto the Peltier plate, which was set at 50 °C. The upper geometry was a 60 mm parallel plate. The measurement was performed at a frequency of 1Hz and at a strain that was within the linear viscoelastic region of the material. After the gel was formed between the parallel plates, a dynamic frequency sweep was conducted at room temperature to compare the mechanical strength of gelatin gels with different concentrations.
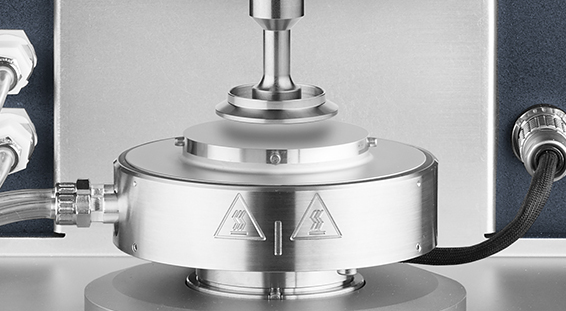
Silicon Based Gel
Silicon based hydrogels are formed through chemical crosslinking reactions. To monitor the gel formation process, the crosslinking agent was added to the silicon monomer solution. The mixture was then loaded onto the Peltier plate, which was set at 37 °C. The upper geometry was a 60 mm parallel plate. A dynamic time sweep was performed at frequency of 1 Hz and a strain that was within the linear region of the material.
Pre-Formed Gels
To study the mechanical properties of a formed hydrogel material, the sample had to be pre-molded or cut into a disk with a smooth and uniform surface. The parallel plate geometry is the preferred geometry for this measurement. Also, an appropriate normal force must be applied to the sample during the test to ensure that the sample is in full contact with the geometry with no slippage. The amount of normal force that is needed is highly dependent on both the flatness and the strength of the gel. If the normal force is too low, the sample will slip during the test. If the normal force is too high, it may damage the gel structure. A Peltier plate immersion cell (shown in Figure 3) can be used to accommodate this requirement.
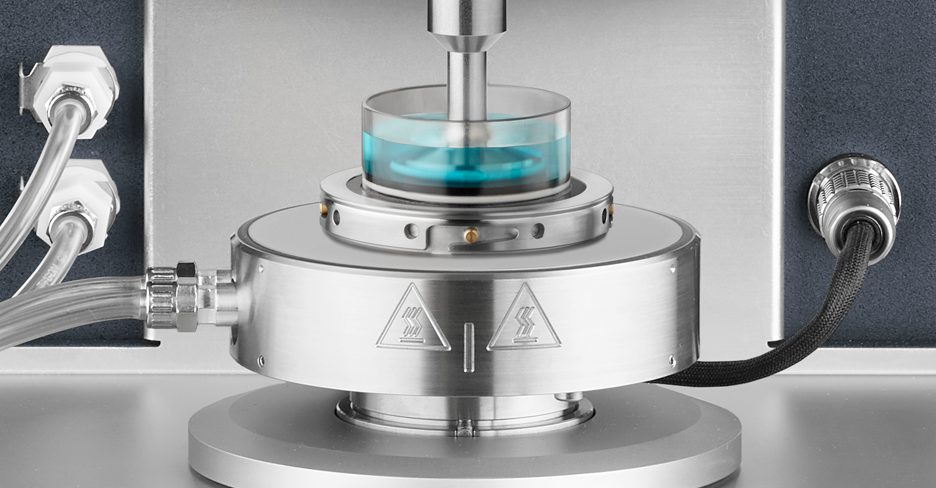
Results and Discussion
A. Monitoring Gel Formation
Gelation As mentioned above, hydrogels can be formed either by a chemical crosslinking or by physical polymer chain association. A rheometer can be used to monitor the gel formation process. Both complex viscosity (η*) and viscoelastic properties (i.e. G’, G”, tan δ) can be quantitatively recorded. Two case studies are provided as examples of monitoring the gel formation of a reversible (physical) and an irreversible (chemical) gel.
Reversible Gel Formation
Gelatin-based hydrogels are formed through polypeptide chain association. Specifically, the peptide chains in gelatin form a triple-helix structure through hydrogen bonding at low temperatures, which leads to the formation of a gel [8].
This kind of gel is thermo-reversible. When heated to a higher temperature, the helical structure dissociates and the gelatin gel “melts” [9]. Figure 4 shows the temperature ramp test results for a 10% gelatin solution at cooling and heating rates of 1 °C/ min and 2 °C/min. Upon cooling, the gelatin solution underwent a liquid-to-solid transition (G’/G” crossover) at temperatures of 22-24 °C. Note that the mechanical strength of the sample (elastic modulus, G’) increased by over five decades during the gelation process. Since the formation of the triple-helix is predominantly a kinetically controlled process, the sol-gel transition (G’/G” crossover) appears at lower temperatures with higher cooling rates. Upon heating, the hydrogel begins to melt at 31 °C. The helical structure totally dissociates, and the polypeptide chains revert to random coils. Clearly, this temperature ramp procedure can be used to monitor the gelation process of a thermally reversible hydrogel.
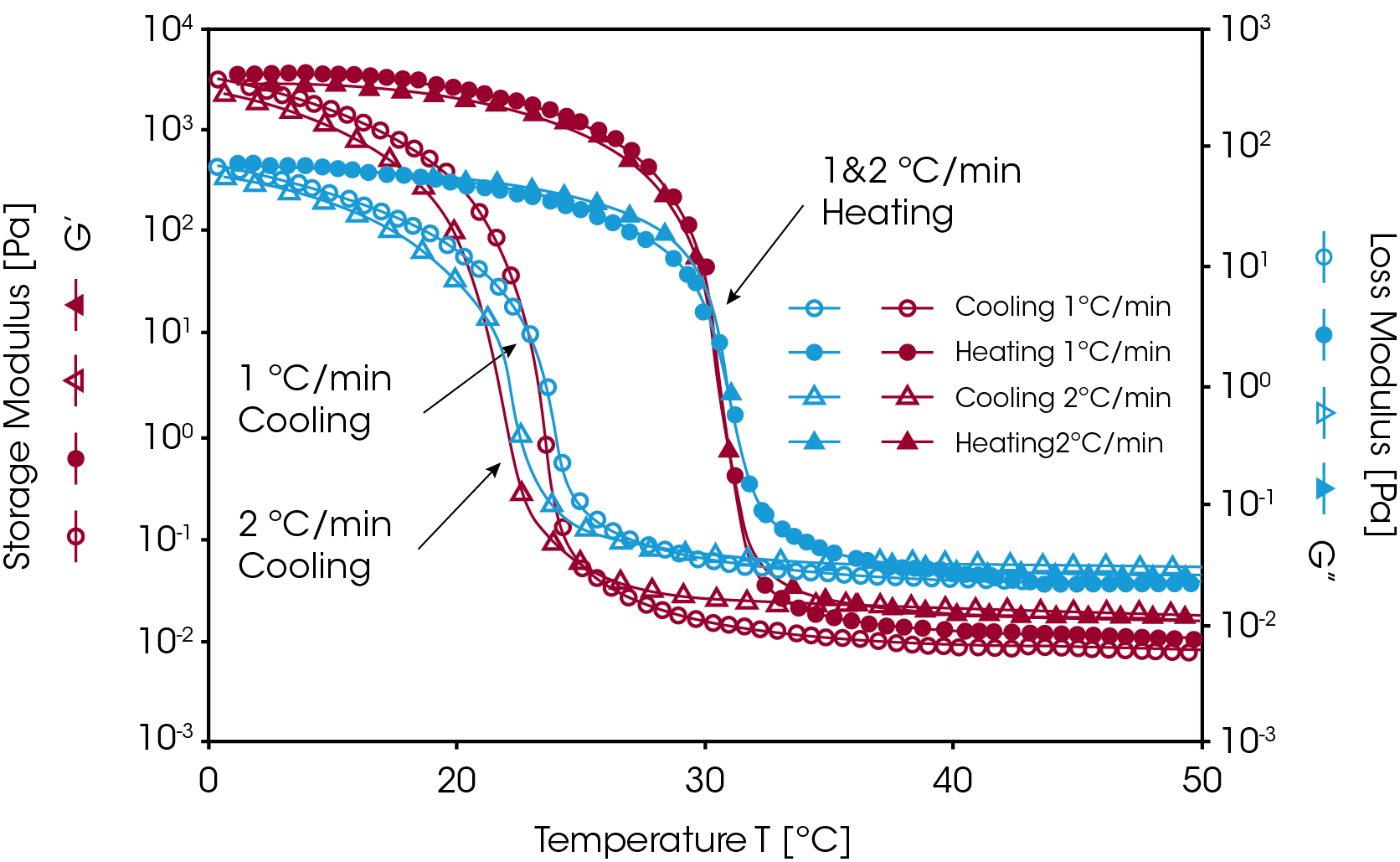
Irreversible Gel Formation
Silicon based hydrogels are commonly used in the biomedical and pharmaceutical industries. These gels are formed through chemical crosslinking reactions. Figure 5 shows an example of monitoring a chemical crosslinking reaction of a silicon-based hydrogel. After mixing the crosslinking agent into the monomer solution, the mixture was loaded onto the Peltier plate. An isothermal dynamic time sweep experiment was conducted at 37 °C. During the measurement, the change in the modulus (G’, G” and G*) and complex viscosity (η*) were monitored as a function of time. The sol-gel transition time (G’/G” crossover time) was also recorded. This transition time can vary depending on the concentration of crosslinking agent and the reaction temperatures. After the gel formation the G’ and G” curves reached plateaus indicating the completion of the crosslinking reaction.
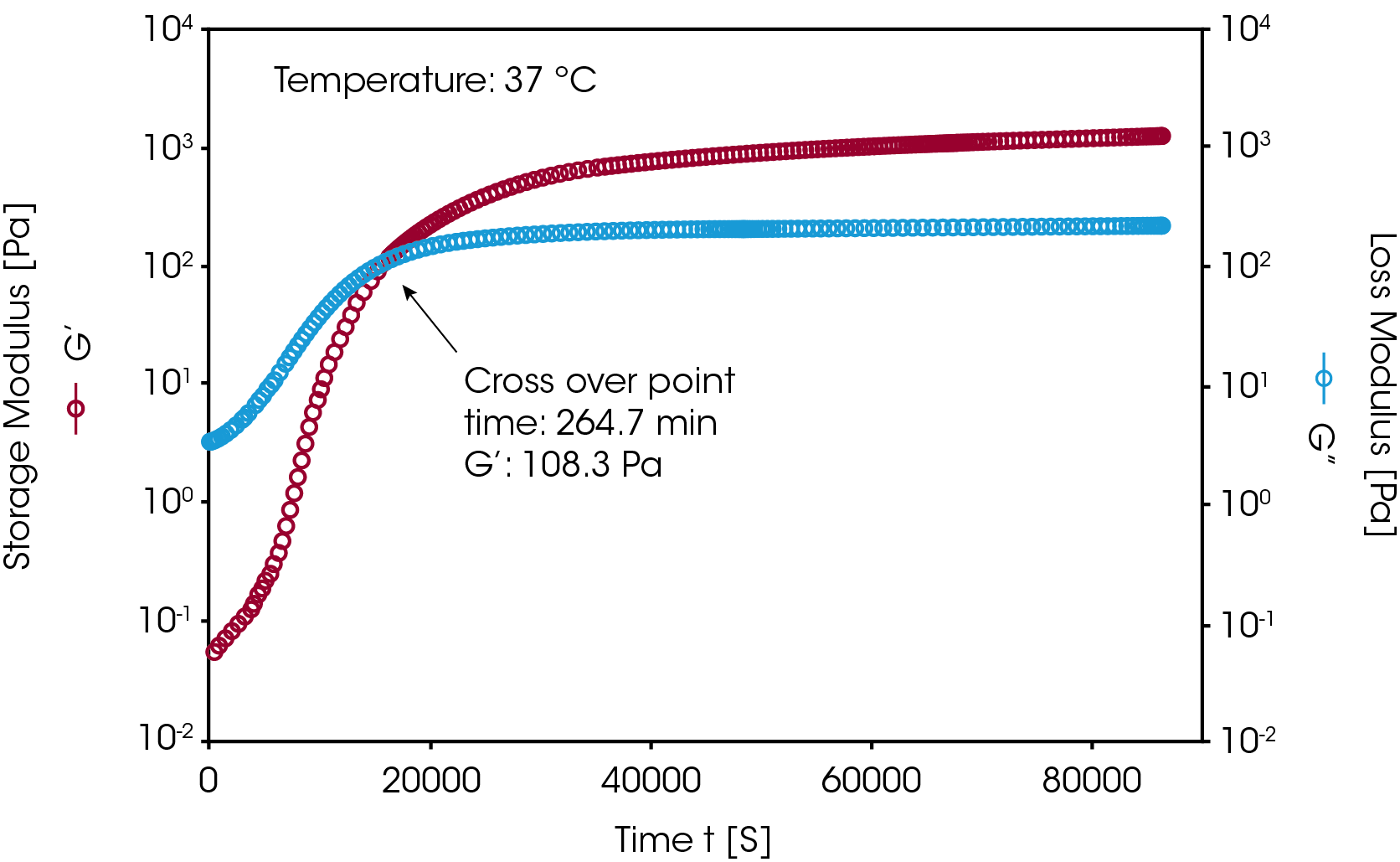
B. Measuring the mechanical properties of a hydrogel
The ideal way to accurately measure the mechanical properties of a hydrogel is to have the gel formed between the geometry plates. This avoids the potential of wall slippage during the test. A gelatin gel is an example of a thermally reversible hydrogel.
In order to measure the mechanical properties of this gel, the sample was loaded onto the Peltier plate at an elevated temperature (50 °C) where the material was in a liquid form. The temperature was then decreased to room temperature and the gel was formed between the plates. Figure 6 shows frequency sweep results of gelatin gels with different gelatin concentrations. No normal force was applied during the measurements. As shown in figure 5, the higher the gelatin concentration, the higher the gel strength (i.e. higher G’). In general, if the moduli curves (G’ and G”) show less frequency dependence, it means the gel has a wellstructured three-dimensional network. The moduli curves for a weaker gel show an increase as test frequencies are increased. The damping factor tan δ can also be used to evaluate the elasticity of a gel. In general, an elastic material shows a lower tan δ value.
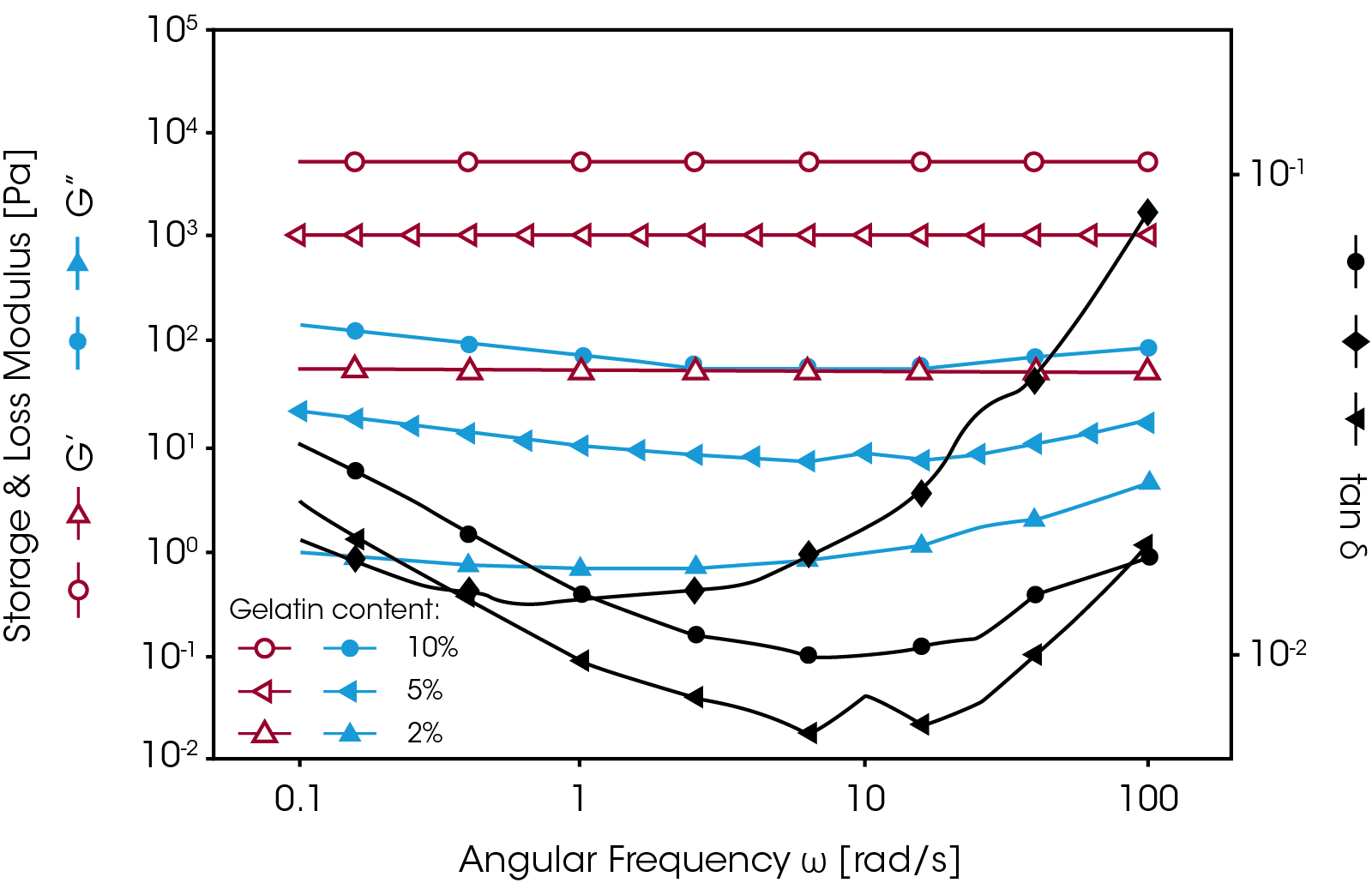
In most cases, however, gels are prepared outside the rheometer. To measure the mechanical properties of this kind of gel, it is necessary to use a crosshatched geometry with appropriate normal force control to avoid sample slippage and to ensure good test accuracy and reproducibility. Figure 7 shows a comparison of the strain sweep results on a silicon-based hydrogel with and without the use of the crosshatched geometry and normal force control. When the standard geometry was used, the sample started to slip at a strain of less than 1%. Upon applying a normal force of 1N, the sample could be held until the strain reached 10%. However, as the normal force was increased, the measured modulus (i.e. G’) increased as well. When testing hydrogels with a normal force applied, the measured modulus is referred to as the apparent modulus since the modulus is dependent on the normal force.
Therefore, in order to get reproducible results, a constant normal force should be applied when studying a series of gels in order to compare their mechanical strength. The crosshatched geometry helped prevent further sample slippage. Figure 7 shows that using the crosshatched geometry, combined with normal force control, provides the most efficient way to prevent wall slip during the measurement.
Some hydrogels must be stored and measured under immersed conditions. Figure 8 shows an example of such an application. A contact lens was tested using the roational rheometer with the Peltier temperature controlled immersion cell (picture shown in figure 3). A dynamic frequency sweep test was performed over a range of eye blinking frequencies (0.5 to 5 Hz). Since a contact lens does not have a uniform thickness it is difficult to measure the absolute modulus. The tan δ value is commonly used for comparing the viscoelastic properties of different contact lens materials. The results show that sample B has better elasticity than sample A and its tan δ value shows less frequency dependence.
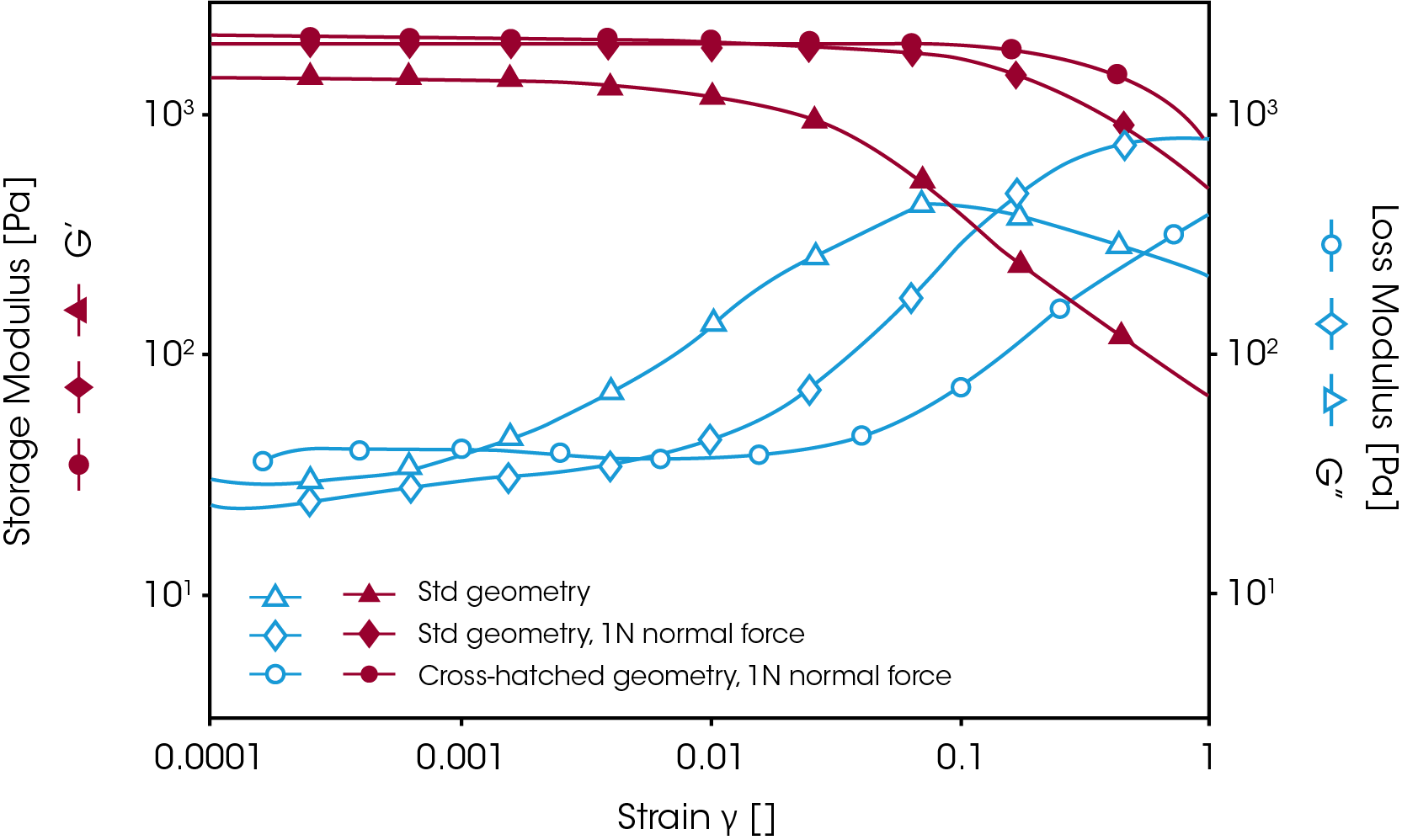
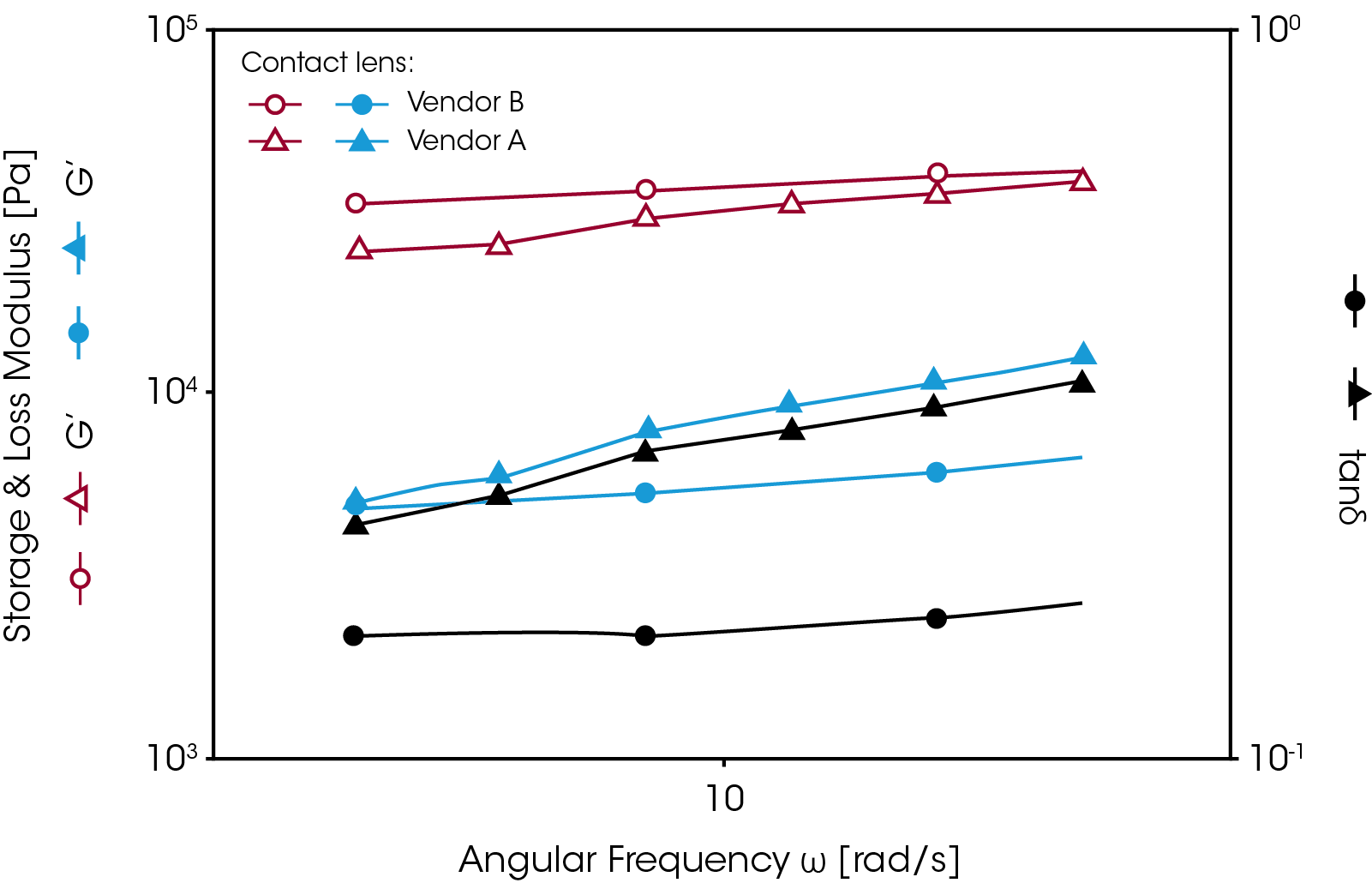
Conclusions
Rheological testing is a powerful technique for studying the viscoelastic properties of hydrogel materials. The gelation process can be monitored by a roational rheometer either isothermally or during a temperature ramp experiment. A frequency sweep test is commonly used to study the mechanical properties of a formed gel. In order to avoid slippage during the measurement, the crosshatched geometry is recommended. Adequate normal force control should also be applied to the gel sample during the measurement. If the sample needs to be measured under immersed conditions, the Peltier immersion cell can be used.
References
- Mani Prabaharan, “Review Paper: Chitosan Derivatives as Promising Materials for Controlled Drug Delivery”. J of Biomaterials Applications, 2008, 23,1,5-36
- Tianhong Chen, David A. Small, Martin K. McDermott, William E. Bentley and Gregory F. Payne. “Enzymatic Methods for In Situ Cell Entrapment and Cell Release”. Biomacromolecules. 2003, 4, 1558-1563 3.
- JH Han, JM Krochta, mj Kurth, YL Hsieh. “Lactitol-based poly(ether polyol) hydrogels for controlled release chemical and drug delivery systems”, J Agric Food Chem. 2000, 48, 11, 5278-82
- Martin K. McDermott, Tianhong Chen, Christina M. Williams, Kolleen M. Markley, Gregory F. Payne. “Mechanical Properties of Biomimetic Tissue Adhesive Based on the Microbial Transglutaminase- Catalyzed Crosslinking of Gelatin”. Biomacromolecules. 2004, 5, 1270-1279
- Tianhong Chen, Rabeea Janjua, Martin K. McDermott, Scott M. Steidl, Gregory F. Payne, “Gelatin-Based Biomimetic Tissue Adhesive for Ophthalmic Applications”. Journal of Biomedical Materials Research Part B: Appl Biomater 77B: 416-422, 2006.
- Vilas D. Athawale, Vidyagauri Lele, “Recent Trends in Hydrogels Based on Starchgraft-Acrylic Acid: A Review”. Starch, 2001, 53, 1, 7-13
- T. Iseki, M. Takahashi,H.Hattori, T. Hatakeyama, and H. Hatakeyama; “Viscoelastic properties of xanthan gum hydrogels annealed in the sol state”. Food Hydrolcolloids, 2001, 15, 4-6, 503-506
- K. H. Mayo, Biopolymers (Peptide Sci), 1996, 40, 359-370
- Payne. “In Vitro Protein- Polysaccharide Conjugation. Tyrosinase- Catalyzed Conjugation of Gelatin and Chitosan”, Biopolymers, 2002, 64, 292-302
Acknowledgement
This note was written by Tianhong (Terri) Chen, Ph.D. Applications Specialist at TA Instruments.
Click here to download the printable version of this application note.