Keywords: microcalorimetry, electrochemistry, lithium-ion batteries, parasitic reactions, hyphenated techniques
Abstract
The Battery Cycler Microcalorimeter Solution integrates TA Instrument’s TAM IV isothermal microcalorimeter with BioLogic’s VSP-300 Potentiostat. This system streamlines the most complex and labor-intensive aspects of battery calorimetry, improving the performance and practical utility of this technology. Control of the potentiostat and calorimeters, import and correlation of both data sets, and automated analysis features are all performed in the integrated TAM Assistant software. The pre-wired battery lifters are calorimeter inserts that house the batteries and facilitate electrical contact to the potentiostat. They are customized to accommodate three common lithium-ion battery designs; 18650 cylindrical cells, pouch cells, and coin cells. This solution is a highly valued instrument for research and development labs and provides greater insights than other current techniques on the market.
Introduction
Traditional research methodologies in battery chemistry have treated materials characterization and thermal analysis as separate from the electrochemical testing. The materials characterization was done to quantify new battery components and how those components changed after cell failure. Thermal analysis was used to design better thermal management systems for end use applications. Most of the information about how the battery performs, what type of chemistry is occurring inside the cell, and how it changes over time, was gathered from the electrochemical data. The information produced from this traditional research workflow left many blind spots in the chemistry [1]. Batteries are highly dynamic systems with a mixture of electrochemical reactions, chemical reactions, and structural changes occurring each cycle. Electrochemical analysis will only give information on processes that affect the electrochemical reactions, leaving all other activities (chemical, phase, structural) uncharacterized. There are many states of change between a new and a failed battery cell and understanding the degradation process requires more insight than current techniques can provide [1,2].
New approaches are focused on combining a secondary analytical technique with electrochemistry to quantify the battery processes during operation. One of the leading strategies towards this effort is the coupling of high resolution isothermal microcalorimetry with electrochemistry [3-6]. Understanding the thermal activity of lithium-ion batteries as a function of the electrochemical stimuli will yield far greater insights than electrochemistry alone. In addition to general thermal management data, calorimetry can also measure parasitic reactions (non-reversible reactions leading to degradation), structural changes, lithium plating, self-discharge rates, and reactions associated with SEI layer growth/decay [3-8]. This data can be used in research to better understand and improve battery formulation chemistry. Battery calorimetry can also be used in quality control to more effectively screen out poorly made cells, owing to its innate compatibility with commercial cell designs.
While electrochemical calorimetry is a powerful tool, the complexity and nuance of the experiments make it difficult to approach for many researchers. Hyphenated or hybrid instrument techniques are commonly plagued with lengthy data processing after the experiment has ended, when correlating the signals from different devices. They also require multiple software interfaces, where the user is forced to synchronize the start times and experiment parameters. The Battery Cycler Microcalorimeter Solution is designed to eliminate these issues and simplify battery calorimetry by integrating the calorimeter and potentiostat at the hardware and software levels.
Battery Cycler Microcalorimeter Solution
The Battery Cycler Microcalorimeter Solution integrates the BioLogic VSP-300 Potentiostat with the TA Instruments TAM IV Isothermal Microcalorimeter (Figure 1). The integrated TAM Assistant software controls both devices, including experiment creation and real time assimilation of the heat flow and electrochemical data. The pre-wired lifters are calorimeter inserts that house the batteries and make electrical contact with the potentiostat. These lifters are designed to conduct heat generated from the battery into the calorimeter, while minimizing noise from ambient thermal fluctuations and wire heating. The connection to the battery is made with a conductive spring clip, so no soldering or additional insulation is required. There are three different lifters to accommodate common lithium-ion battery dimensions. The coin cell lifter will house a coin (button) cell up to 23 mm in diameter. This lifter is compatible with any 20 mL calorimeter on the TAM IV, including the Microcalorimeter and Multicalorimeter (3-pack) configuration. The Macrocalorimeter lifter fits standard 18650 size cylindrical cells, most commonly used for electric vehicles. The Micro-XL lifter will house pouch cells with maximum dimensions of 50 mm width, 94 mm length, and 12 mm thick. The tabs are held by an adjustable clamp (with conductive contacts) to accommodate a variety of tab spacings.
Depending on the testing needs, the TAM IV calorimeters can be configured in several different ways to maximize throughput. If the TAM IV is fitted with 4 Multicalorimeters, and two VSP-300 Potentiostats (housing 6 channels each), a user can test 12-coin cells simultaneously in discrete experiments.
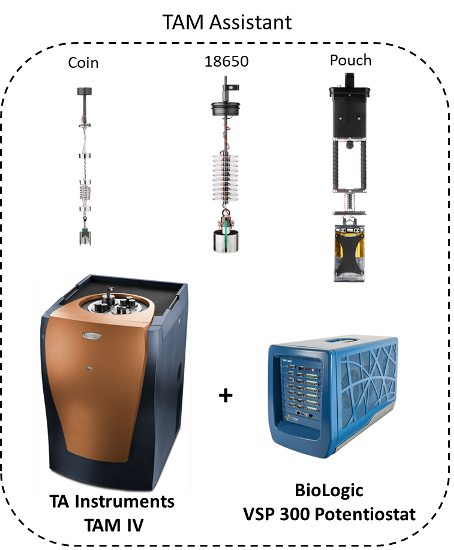
Integrated Software
From a workflow perspective, the most important aspect of the battery cycler microcalorimeter is the software integration between the TAM IV and the VSP-300. TAM Assistant can control the TAM IV and the VSP-300 Potentiostat simultaneously to facilitate battery calorimetry experiments from a single software interface. The new experiment wizards for battery experiments are shown in Figure 2. The experiment wizard will guide the user through selecting a method, customizing parameters, loading the sample, and ensuring proper baseline stability.
An overview of available methods is given below.
- Manual: Custom experiments, programmed by selecting actions, wait times, and event markers in the run sequence menu.
- Heat management (CCCV): Measures heat flow during cycling, primarily used for thermal management applications. This method will program different charging profiles (variable charge / discharge rates) using constant current constant voltage (CCCV) parameters.
- Entropy changes: This method consists of a slow cycle (C/20) followed by a very slow cycle (C/100) for maximum resolution into the structural and phase changes that occur during lithiation/delithiation.
- First cycle reaction (SEI): Method designed to study the SEI formation reactions. This method applies a small charging current in the low voltage range where SEI formation occurs (typically below 3.0 V), then applies a higher charging current until the standard upper voltage limit is reached (typically 4.2 V).
- Full cycle parasitics: Method to measure the parasitic power over the full voltage range. The term Parasitics is a blanket term for any non-reversible side reaction that contributes to battery degradation.
- Narrow cycle parasitics: Method to measure the parasitic power in a narrow voltage. This is a faster technique if only one (or more) narrow voltage windows are of interest.
- Self-discharge: Experiment for measuring the self-discharge rate. This combines the conventional technique (monitoring open circuit voltage over time, then discharging to measure the remaining capacity) and the thermal method of integrating the total heat produced over the open circuit time.
During the experiment, the thermal and electrochemical signals are plotted in the same window (Figure 3). In order for the data to be meaningful, it is correlated with an unmatched high degree of precision. Both sets of data are timestamped and automatically correlated by the software during import. This is a significant improvement over manual data processing, which takes much longer and often has transcription errors.
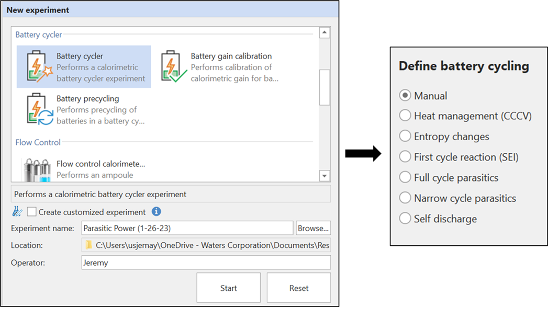
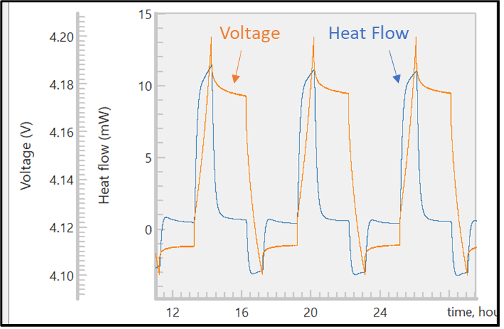
Analysis Tools
After the experiment has concluded, there are several analysis options in the results file. The integrated TAM Assistant software will automatically calculate key values and present them in a table or as a plot. There are several options for the x-axis and y-axis, the ability to overlay multiple cycles, and the option to separate charge from discharge (see Figure 4). These tools were designed to maximize flexibility, speed, and ease of use, so the user could more efficiently find trends or features within the data. The summary table presents the averaged or accumulated signals, including coulombic efficiency and average parasitic power. More information regarding the calculations, theory and calibration can be found in the TA Instruments application note entitled “Determination of Parasitic Power in Lithium-Ion Batteries using the Battery Cycler Microcalorimeter Solution.”
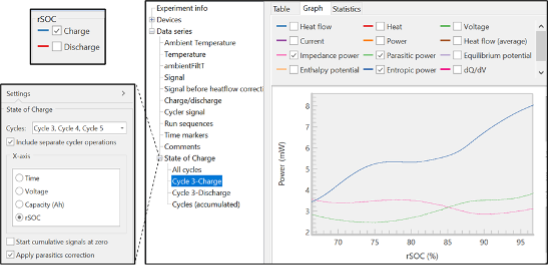
Conclusions
Calorimetry of full battery cells is a valuable tool for thermal management, electrolyte additive research, active material formulation, and quality control. The barrier to this technology was the complexity of the experiments and labor-intensive data processing. The Battery Cycler Microcalorimeter Solution integrates the TAM IV with the VSP-300 at the hardware and software levels to improve accessibility, reliability, and the general utility of battery calorimetry.
References
-
- Liu D, Shadike Z, Lin R, Qian K, Li H, Li K, Wang S, Yu Q, Liu M, Ganapathy S, Qin X, Yang QH, Wagemaker M, Kang F, Yang XQ, Li B. Review of Recent Development of In Situ/Operando Characterization Techniques for Lithium Battery Research. Adv Mater. 2019, 28, 1806620.
- Matthew G. Boebinger, John A. Lewis, Stephanie E. Sandoval, and Matthew T. McDowell. Understanding Transformations in Battery Materials Using in Situ and Operando Experiments: Progress and Outlook. ACS Energy Letters,2020,5 (1), 335-345.
- L.J. Krouse, L.D. Jensen, J.R. Dahn. Measurement of Parasitic Reactions in Li Ion Cells by Electrochemical Calorimetry. J. Electrochem. Soc. 2012, 159 (7), A937-A943.
- L.E. Downie, S.R. Hyatt, J.R. Dahn. The Impact of Electrolyte Composition on Parasitic Reactions in Lithium Ion Cells Charged to 4.7 V Determined Using Isothermal Microcalorimetry. J. Electrochem. Soc. 2016, 163 (2), A35-A42.
- L.J. Krouse, L.D. Jensen, V.L. Chevrier. Measurement of Li-Ion Battery Electrolyte Stability by Electrochemical Calorimetry. J. Electrochem. Soc. 2017, 164 (4), A889-A896.
- Alexander Kunz, Clara Berg, Franzika Friedrich, Hubert A. Gasteiger, Andreas Jossen. Time-Resolved Electrochemical Heat Flow Calorimetry for the Analysis of Highly Dynamic Processes in Lithium-Ion Batteries. J. Electrochem. Soc. 2022, 169, 080513.
- J.C. Burns, Adil Kassam, N.N. Sinha, L.E. Downie, Lucie Solnickova, B.M. Way, J.R. Dahn. Predicting and Extending the Lifetime of Li-Ion Batteries. J. Electrochem. Soc. 2013, 160, A1451.
- Downie, Laura, Krause, L., Burns, J, Jensen, L, Chevrier, V, Dahn, J. In Situ Detection of Lithium Plating on Graphite Electrodes by Electrochemical Calorimetry. Journal of The Electrochemical Society. 2013, 160, A588-A594.
Acknowledgement
This paper was written by Jeremy May, PhD at TA Instruments
Click here to download the printable version of this application note.