Keywords: Microcalorimeters, Micorcalorimetry, Isothermal Calorimetry, API, Excipient
MC167
Background
Development of new pharmaceutical products is complex and time-consuming, and new therapeutic drugs must meet specific testing standards to ensure their safety, efficacy, and quality. Identifying the physical and chemical incompatibilities between active pharmaceutical ingredients (API) and excipients during early phase-development is an essential step to maintain the target products critical to quality attributes throughout the clinical process. Isothermal microcalorimetry is an alternative to the conventional test methods that enhances detection of incompatibilities by significantly reducing testing time, improving testing throughput, and reducing operator effort.
Excipient Compatibility by HPLC
HPLC analysis is the most common method used to study API/excipient blends for incompatibilities (Higgins et al., 2003). Mixtures of the API and excipient are studied at elevated temperatures, and often at elevated humidity, in order to enhance reactivity; however, these studies can require weeks until enough API has reacted to allow conclusions about incompatibilities to be drawn. This approach can also be labor intensive: for example, samples must be periodically removed for analysis throughout the study, and mobile phase extractions or filtrations may be required before injection into the HPLC if solubility problems are encountered.
Excipient Compatibility by Isothermal Microcalorimetry
Isothermal microcalorimetry (IMC) offers the formulator a simple and less time-consuming alternative for determining if chemical and/or physical interactions can be expected between the API and excipients. These studies can be carried out in early and/or late phase development. Excipients showing promise after screening by microcalorimetry can then be studied using more traditional methods (HPLC), resulting in potentially significantly less time and effort compared to using conventional methods to screen all the excipients (Phipps et al., 2000).
In a typical compatibility experiment, a solution, suspension or solid mixture of the API and excipient is placed in the calorimeter and the thermal activity (heat flow) at a constant temperature is monitored. The basic assumption is that the rate of heat production is proportional to the rate of chemical and/or physical processes taking place in the sample.
A simple example would be the conversion of A to B:
A Δ B
Thermal activity = dq/dt = ΔH(dn/dt)
where q is the heat (e.g., calories), t is time in seconds, ΔH is the heat of reaction, and n is the number of moles of B formed.
Therefore, the output of the calorimeter is directly proportional to the rate of reaction or conversion at any given time t. The rate of heat production is proportional to the rate of chemical and/or physical processes taking place in the sample.
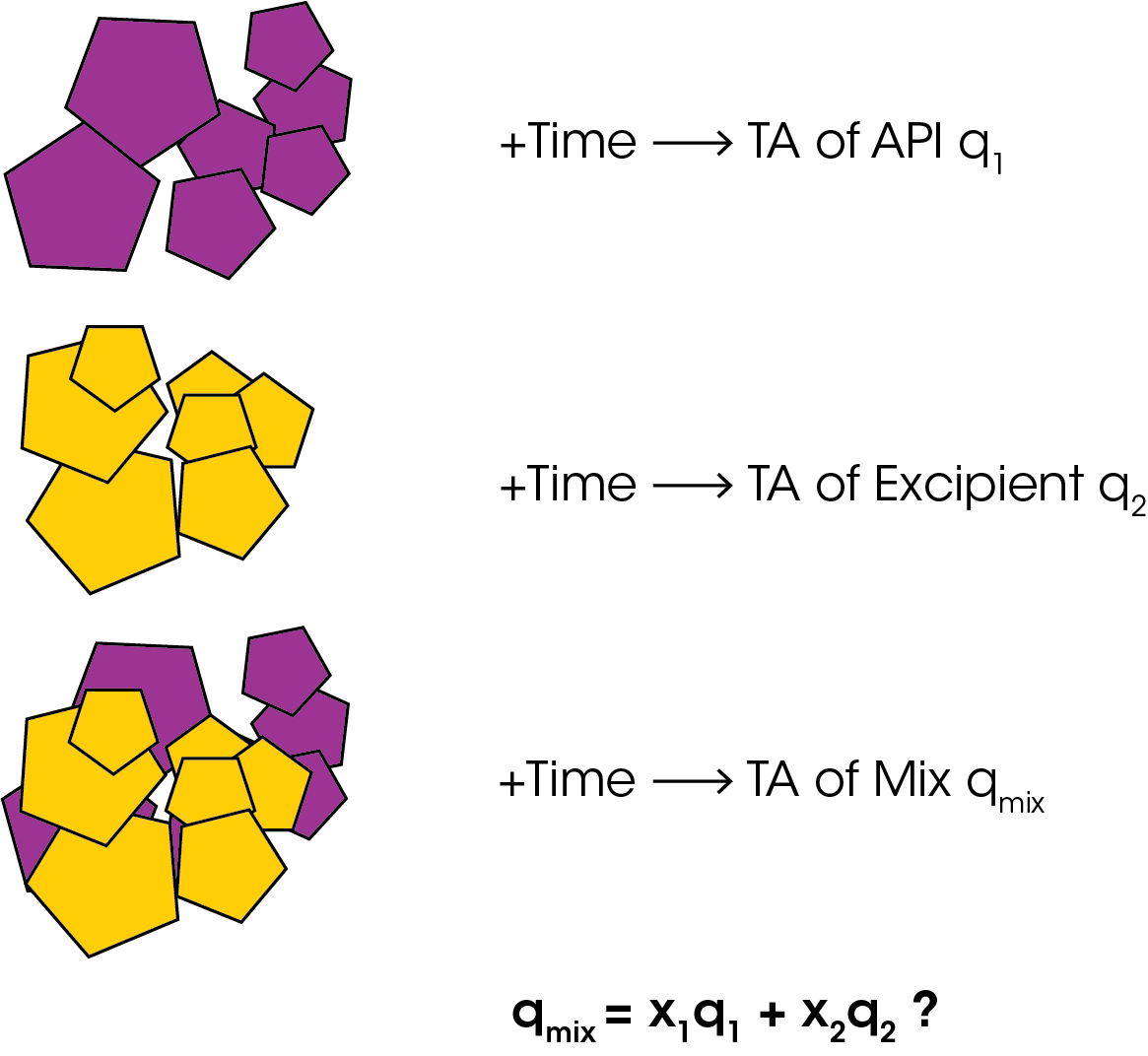
The rate of heat produced by the API and excipient are measured individually, and the sum of the heat rates from the individual components is compared with the heat rate from the mixture. If the heat rate from the mixture is significantly different from the sum of the heat rates from the individual components, the excipient is incompatible with the API.
Because the signal may be the sum of numerous chemical and physical processes, usually no attempt is made to correlate the signal with the rate of degradation. Rather the method is intended to be an indicator of potential incompatibility. This reduces the number of samples that must be studied by slower, conventional methods, saving valuable time and effort during the formulation process.
Figure 2 shows an example where the heat out put of the API/excipient blend matched the sum of the heat output from the API plus excipient: Notice that the difference between the sum of the curves from the individual components and the curve for the API/excipient blend is less than the sample-to-sample variation of the API and excipient (usually estimated from historical data on a given excipient). There can be significant differences in heat output from one sampling to the next within a single batch of API or excipient, hence a range of values reflecting the variation in API and excipient reaction rates must be used as part of the criteria for determining compatibility vs. incompatibility.
Figure 3 shows the same API mixed with a different binder where clearly there is evidence of an incompatibility: Since the observed heat rate depends on the enthalpy change for the reactions, as well as the rate of reaction, generally no attempt is made to correlate the signal with “how much” or “how fast” any process is taking place. This is because a small but significant signal could in fact be due to a rapid physical change with a small enthalpy, for example an excipient acting as a nucleation site, resulting in enhanced polymorph conversion. On the other hand, the heat change could be due to a very exothermic chemical reaction, such as oxidation, occurring very slowly. Therefore, the “pass” or “fail” criterium is based on the simple observation if a “chemical or physical process was detected” versus “no chemical or physical process was detected”.
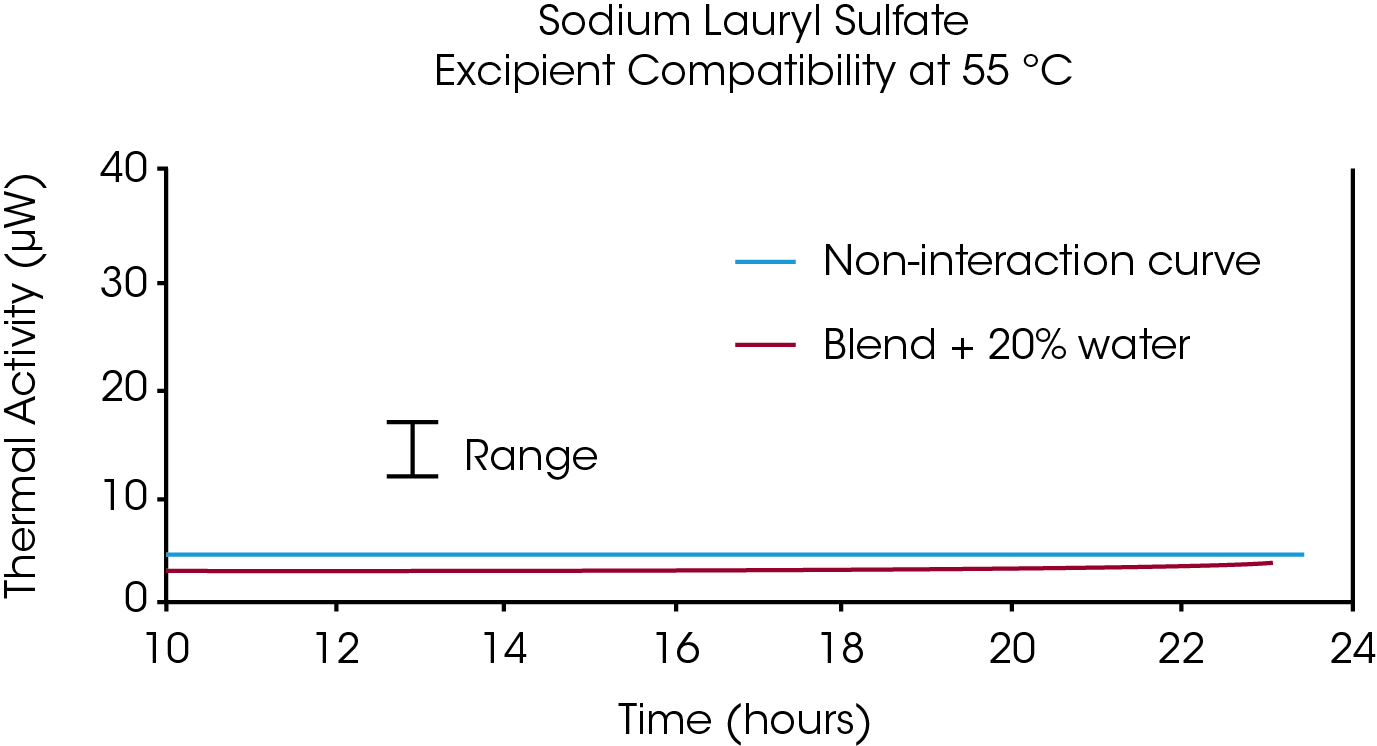
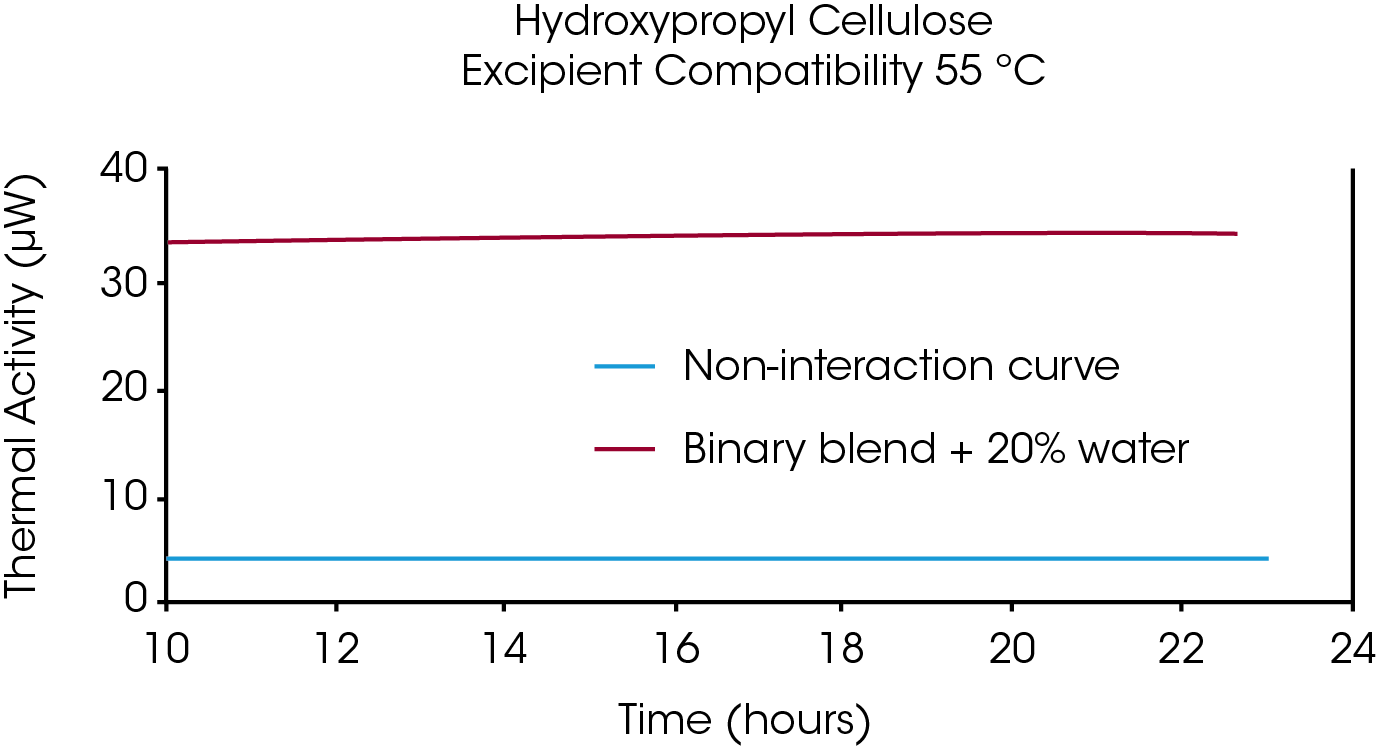
Instrumentation
Modern isothermal microcalorimeters can measure extremely small heat flows. Based on assumptions about molecular weight, enthalpy and reaction mechanism, degradation rates of less than 1% per year can be detected (Angberg et al., 1995), although it is important to remember that the observed thermal activity can be due to a number of processes, and not only a chemical reaction. Other processes that can be detected include conversion of amorphous to crystalline material, polymorph conversions, dissolution processes, etc. Also, within a single batch of API or excipient, significant differences can be observed in heat output from one sampling to the next (hence the use of a range of values as part of the criteria for determining compatibility versus incompatibility).
Experiments are usually run for 24 to 48 hours, with the idea that non-chemical processes will come to completion (e.g., dissolution) early in the experiment. Typically, if any chemical reaction(s) are occurring, these events are followed by an output consistent with pseudo zero order degradation. Schmitt et al. (2001) recommends adding 20% water to enhance reactivity and running isothermally at 50 °C, similar to the HPLC method mentioned previously.
The output from a microcalorimeter can be amplified by performing experiments at elevated temperatures. Scanning microcalorimeters (also known as HSDSC for “high sensitivity differential scanning calorimeter”), while less sensitive than dedicated isothermal systems, can be programmed to step through a number of temperatures in a single run. This makes it possible to study compatibility starting at modest temperatures (e.g., 35 °C) and ending at very high levels of thermal stress (e.g., 85 °C) on the same sample in a single 24– 48 hour run.
Graphing the log (heat flow) versus the reciprocal temperature results in a classical Arrhenius plot, from which the temperature where apparent non-Arrhenius behavior begins can be determined. This can be very useful information when long-term stability testing begins in the later phases of the development process.
Practical Examples
The following examples serve to illustrate how microcalorimetry can be used to quickly screen excipients during the formulation process.
Example 1: The Maillard Reaction
A common incompatibility encountered by pharmaceutical formulation groups is between lactose (anhydrous or monohydrate) with APIs containing primary or secondary amines. Figure 4 shows how this common incompatibility, known as the Maillard reaction, can easily be detected using microcalorimetry.
The red trace represents the calculated combined heat output from the API and lactose (100 mg solids), analyzed separately by IMC. All samples contained 20% water, as suggested by Schmitt et al. (2001). The large exothermic process during the first 10-12 hours appeared to level off to a pseudo first order reaction after about 14 hours. The initial exothermic process is likely due to a combination of the Maillard condensation reaction and other processes such as the conversion of amorphous to crystalline material, the formation of hydrates, etc. As mentioned previously, these processes are assumed to come to completion rapidly compared to the degradation of the API, which can take weeks or longer to complete. Therefore, the data in Figure 4 are consistent with a chemical interaction resulting in incompatibility between the excipient and the API.
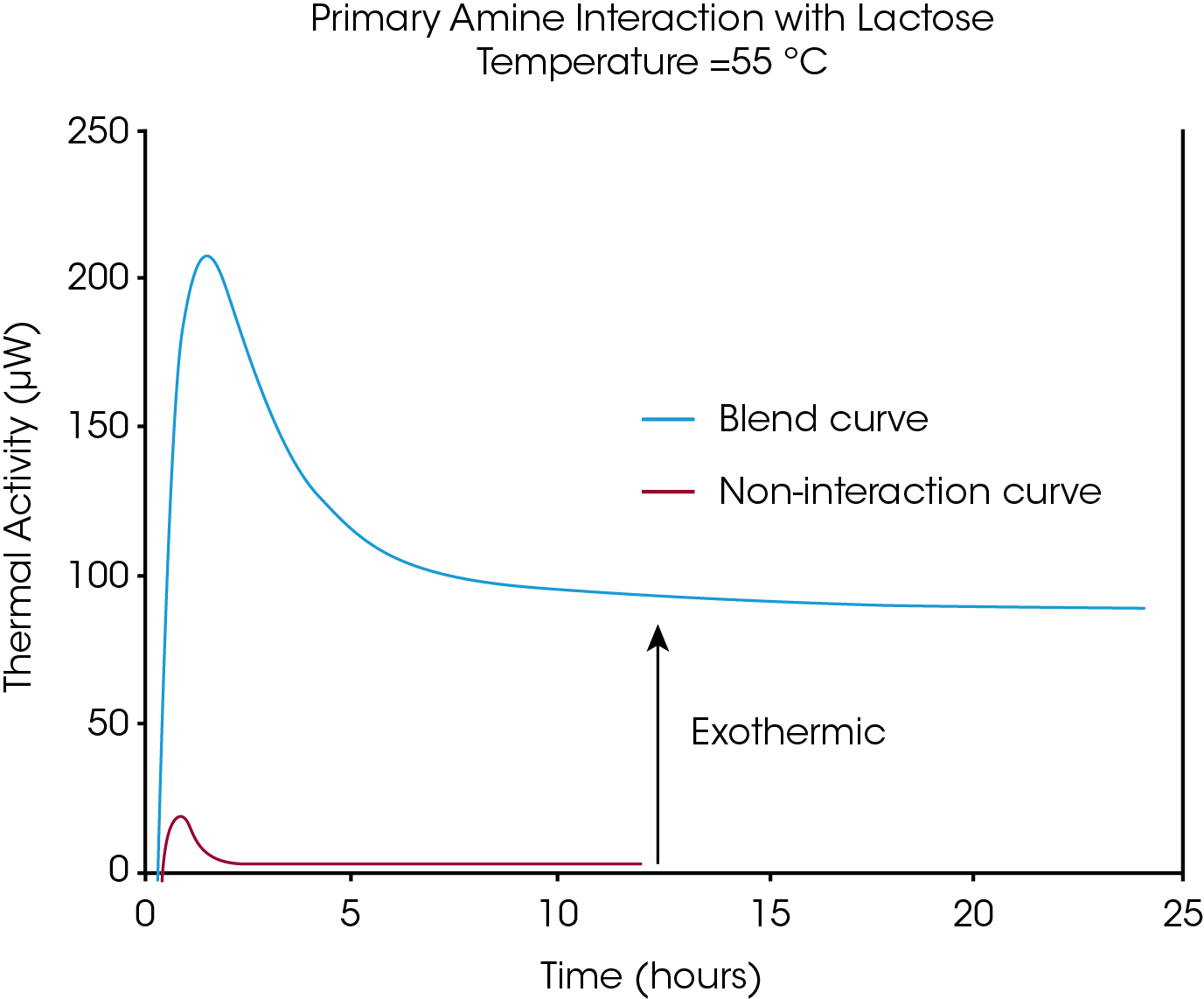
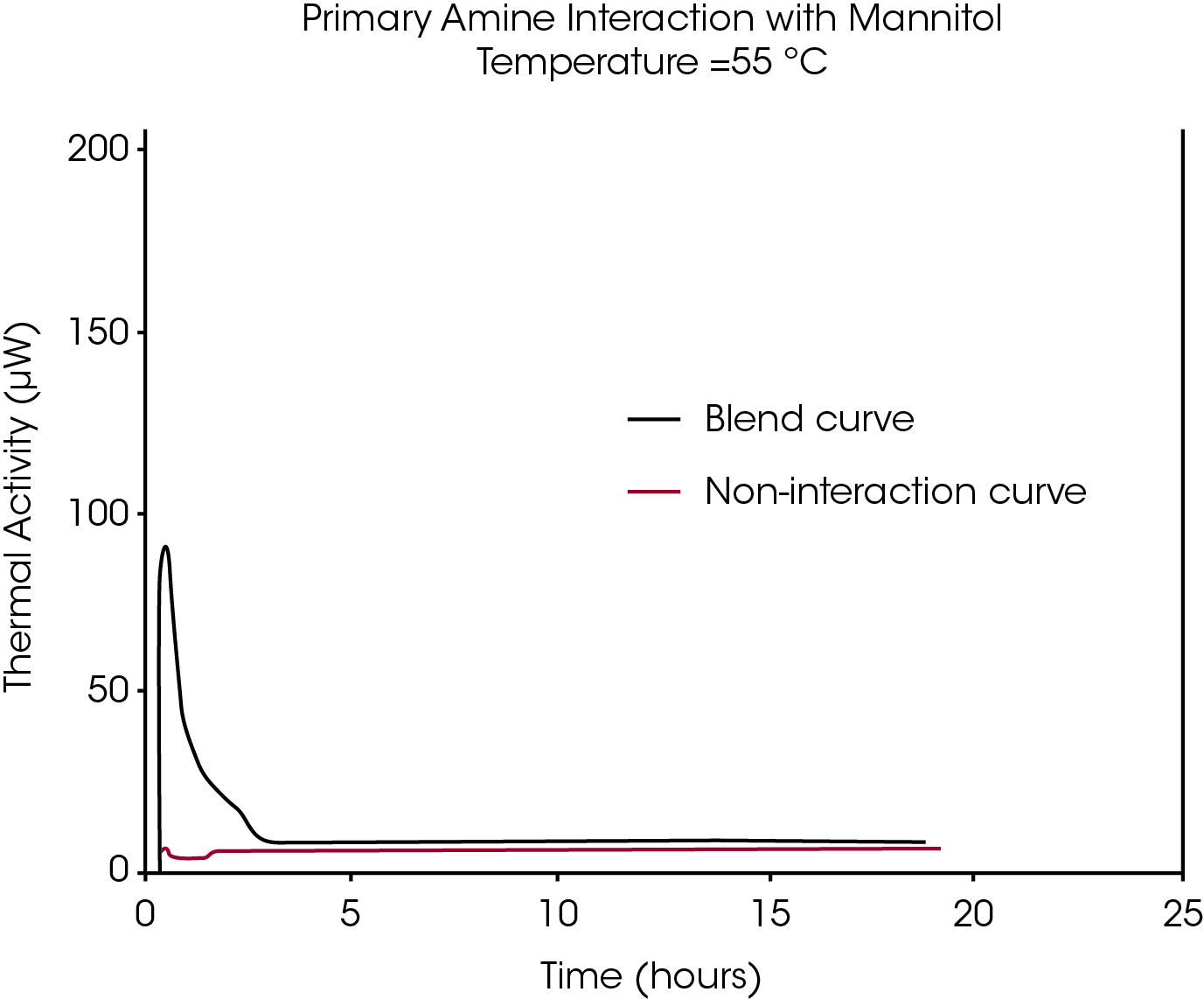
Example 2: Compatible Alternative for Lactose
Figure 5 shows data for the same API, but mixed with mannitol. The API/mannitol blend does not show any significant difference compared to the sum of the curves for the individual components, suggesting that mannitol is a promising alternative to lactose in a solid dosage formulation of this API.
Example 3: Solution Compatibility
Many pharmaceutical compounds are formulated as solutions. Microcalorimetry can be used to screen excipients in these systems in a similar manner to that used for solid dosage formulations. Figure 6 illustrates that lactose has no apparent net influence on the degradation of the antibiotic at 90 °C. High temperature was used to accelerate the degradation of the API in order to obtain a large signal. Additional examples from the literature can be found in the quoted and general references.
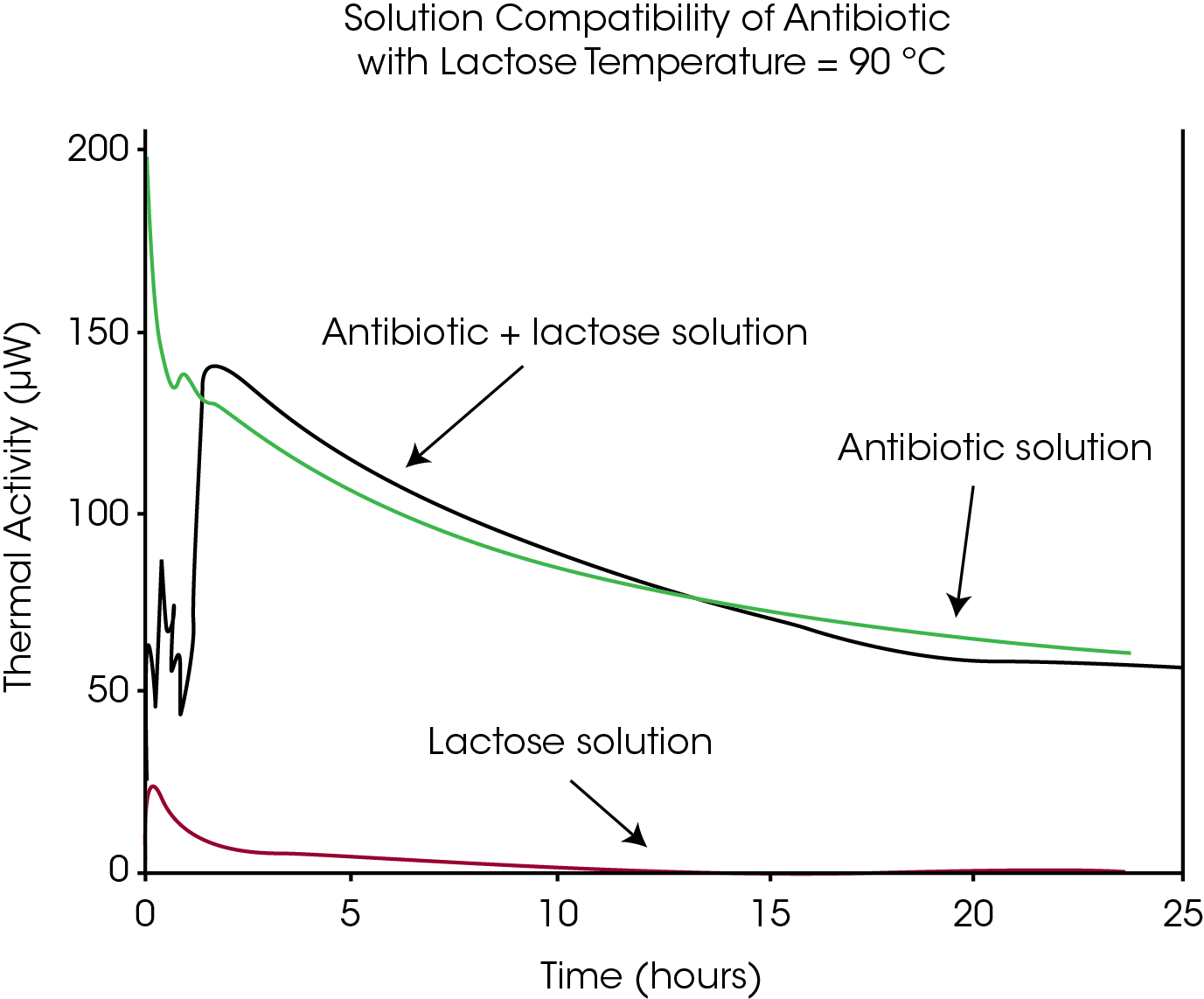
Summary
Microcalorimetry offers a rapid and easy approach for screening excipients for use in solid and liquid formulations. The experimental method is simple and can be applied to any sample, eliminating the need to develop a robust HPLC method before compatibility studies on a drug candidate can begin. A single experiment screens for both physical and chemical interactions, eliminating the need to use expensive, time-consuming methods such as X-ray powder diffraction to monitor physical processes (for example, the conversion of the drug from crystalline to amorphous form).
By quickly eliminating excipients which are obviously incompatible with the API, only those with good prospects for performing well in formulations would be tested using more traditional methods such as HPLC. This would save significant time and effort, compared to testing all possible excipients using more labor-intensive methods. Late in formulation development, problems may arise (e.g., compressibility, dissolution rate) that require replacement of an excipient. Microcalorimetry is a very powerful tool in these cases since numerous potential replacements for binders or diluents can be quickly screened, and then tested in the formulation.
The same process can also be used to quickly screen potential alternatives to excipients if post-formulation chemical or physical development stability issues arise. Manufacturing changes and their effect on compatibility can also be screened with minimal effort. Common examples would be when suppliers of an excipient change their manufacturing processes, or when cost or capacity issues require that a different vendor be chosen to supply an excipient.
References
- Angberg, M. (1995) Lactose and thermal analysis with special emphasis on microcalorimetry. Thermochim. Acta 248, 161–176.
- Buckton, G., S. J. Russell and A. E. Beezer. (1991) Pharmaceutical calorimetry: a selective review. Thermochem. Acta 193, 195-214.
- Chadha, R., N. Kashid and D. V. S. Jain. (2004) Evaluation of the in vitro compatibility of amoxicillin/clavulanic acid and ampicillin/sulbactam with ciprofloxacin. J. Pharmaceut. Biomed. Anal. 36, 295-307.
- Gaisford, S. (2005) Stability assessment of pharmaceuticals and biopharmaceuticals by isothermal calorimetry. Curr. Pharmaceut. Biotech. 6, 181-191.
- Gaisford, S. and G. Buckton. (2001) Potential application of microcalorimetry for the study of physical processes in pharmaceuticals. Thermochem. Acta 380, 185-198.
- Higgins, J. D. et al. (2003) A stop along the drug development highway. Today’s Chemist at Work. July issue. 22–26.
- Phipps, M. A. et al. (2000) Application of isothermal microcalorimetry in solid state drug development. Pharmaceut. Sci. Technol. Today 3, 9–17.
- Royall, P. G. and S. Gaisford. (2005) Application of solution calorimetry in pharmaceutical and biopharmaceutical research. Curr. Pharmaceut. Biotech. 6, 215-222.
- Schmitt, E. A. et al. (2001) Rapid, practical and predictive excipient compatibility screening using isothermal microcalorimetry. Thermochim. Acta 380, 175–183.
- Selzer, T., M. Radau and J. Kreuter. (1998) Use of isothermal heat conduction microcalorimetry to evaluate stability and excipient compatibility of a solid drug. Int. J. Pharm. 17, 227-241.
- Serajuddin, A. T. M. et al. (1998) Compatibility testing. J. Pharmaceut. Sci. 8, 696– 704.
- Skaria, C.V., S. Gaisford, M. A. A. O’Neill, G. Buckton and A. E. Beezer. (2005) Stability assessment of pharmaceuticals by isothermal calorimetry: two component systems. Int. J. Pharm. 292, 127-135.
- Thompson, K.C. (2000) Pharmaceutical applications of calorimetric measurements in the new millennium. Thermochim. Acta 355, 83-87.
- Waterman, K. C. and R. C. Adami. (2005) Accelerated aging: Prediction of chemical stability of pharmaceuticals. Int. J. Pharm. 293, 101-125.
- Wang, W. (1999) Instability, stabilization and formulation of liquid protein pharmaceuticals. Int. J. Pharm. 185, 129-188.
- Wissing, S., S. Craig, S. A Barker and W. D. Moore. (2000) An investigation into the use of stepwise isothermal high sensitivity DSC as a means of detecting drug excipient incompatibility. Int. J. Pharm. 199, 141-150.
Acknowledgement
Click here to download the printable version of this application note.