Rheology of Hydrogels
Sarah Cotts | Morgan Ulrich
June 20, 2022
Characterizing and Applying Hydrogels
Hydrogels are three-dimensional porous structures that can absorb large amounts of water. They can be made up of polymers, protein, peptides, colloids, surfactants, or lipids.1 Hydrogels’ ability to uptake large amounts of water is useful for many biological applications, including drug delivery and tissue engineering. Since a hydrogels’ properties change as it absorbs water, scientists must accurately characterize its behavior at different saturation amounts and in varying conditions.
An example of a naturally occurring hydrogel is collagen, the main structural protein in the human body. Collagen is an ideal material for tissue structures due to its fibrous nature – its molecules are tightly packed together in long fibrils and, depending on the exact structural arrangement, can produce fibrils with similar tensile strengths to steel but with some flexibility.
Collagen’s remarkable structural properties and biocompatibility lead to it becoming a widely used material, particularly as a scaffold in tissue engineering.2 Since collagen is a natural material, its qualities vary from sample to sample. Therefore it can be challenging to manufacture collagen-based products with reproducible properties. However, the inherent variability of natural starting materials is not an insurmountable challenge – it simply requires the correct material property testing to be performed to predict the material’s behavior for the final application.
Throughout this blog we will use collagen as our primary example of a hydrogel to characterize. However, these same techniques can be applied to any hydrogel of any source material.
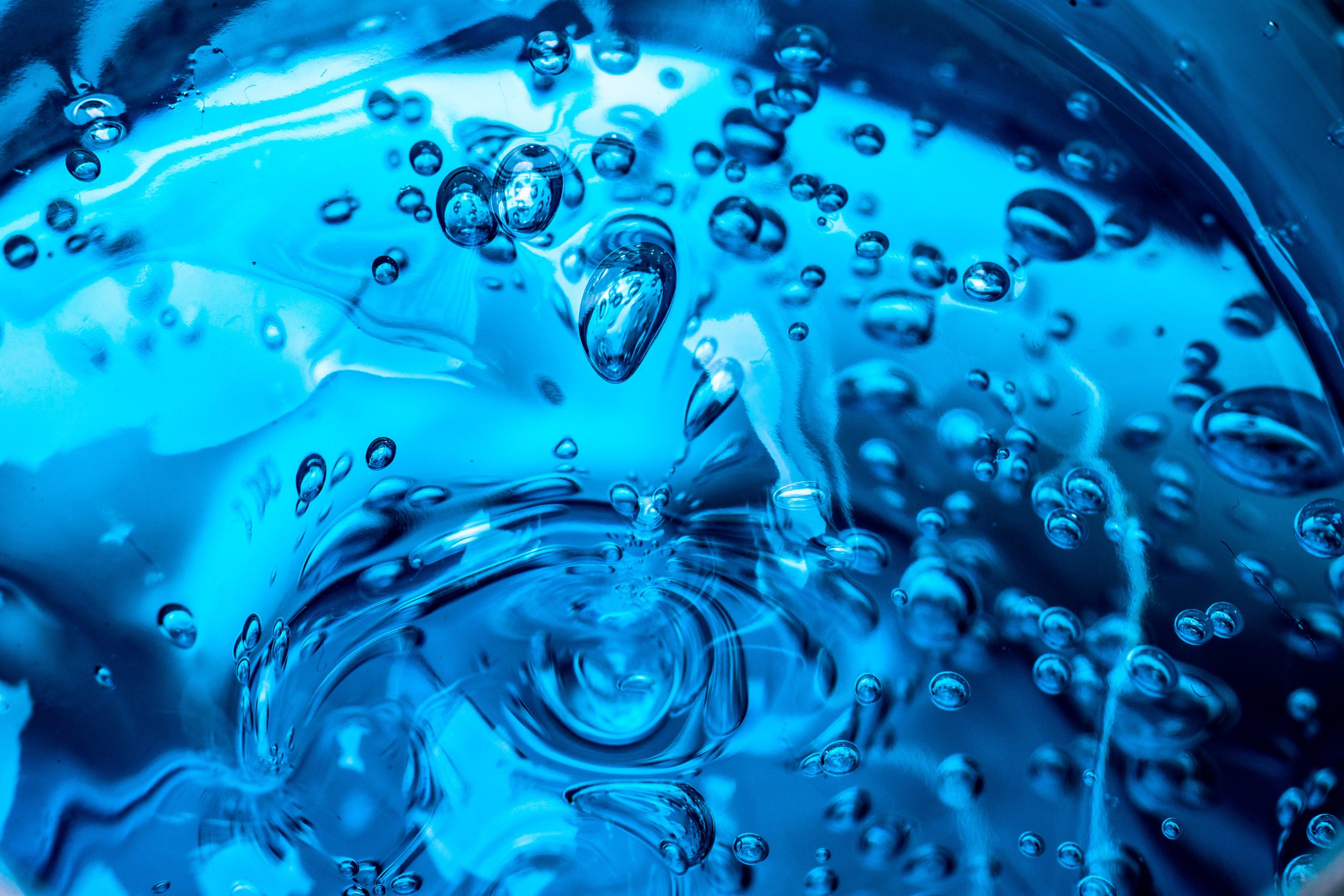
Rheology of Hydrogels
Rheology – the measure of flow and deformation behavior of liquids and solids – is an ideal methodology for studying hydrogels such as collagen. Hydrogels are inherently viscoelastic, meaning they exhibit both viscous and elastic behavior. Dynamic (oscillatory) rheology characterizes these complex materials and reports quantitative measurements of viscoelasticity:
- Storage modulus: how much energy must be applied to distort the sample
- Loss modulus: the energy lost as the material returns to its original shape after deformation
- Tan delta: the ratio of the loss modulus to the storage modulus and the measure of the damping abilities of the hydrogel
These viscoelasticity measurements are critical when trying to understand the differences in the degree of cross-linking, glass transition, heterogeneity, and molecular weight between hydrogel samples and how it impacts the end-use performance.1 Rheometers easily measure viscoelasticity and are the ideal tool for optimizing these qualities.
Another important measurement that is critical for hydrogels is yield stress. A lot of hydrogels are injectable materials so yield stress is very important as it determines how the hydrogel is retained at the injection point. The shear response also determines how easy the hydrogel solution is to inject.
Since different collagen starting materials can have very different physical and thermal properties, researchers must carefully characterize collagen under realistic application conditions to select the best materials for specific uses.3 Rheology test equipment’s rapid screening capabilities makes industrial collagen processing both efficient and cost-effective.
Choosing the Best Rheometer for Hydrogels
Performing high-quality, reproducible rheology measurements requires the right test instrument. TA Instruments is a world leader in the design and manufacture of test systems for materials properties, including for rheology.
Our signature rheometer, the Discovery Hybrid Rheometer, is an industry-leading instrument for rheological measurements. The easy-to-use DHR performs a wide range of standard and advanced analysis measurements that are crucial for hydrogel characterization.
The Discovery HR
The Discovery HR can perform several standard operating procedures for the quality control of collagen hydrogels, including the evaluation of viscoelasticity, including evaluating under which stress conditions the deformation of the hydrogel starts to become nonelastic.4 For hydrogels this type of evaluation is particularly important, as samples that exhibit such viscoelastic properties at very low strain percentages will not be suitable for the final product. The Discovery HR Rheometer makes it possible to rapidly identify candidates best suited for specific medical applications.
The Discovery Hybrid Rheometer is designed for ease of use across simple and advanced measurements. The instrument is also compatible with the TRIOS AutoPilot software to create automated routines, including measurement, analysis and report generation. For industrial manufacturing, where time is of the essence, this software can help accelerate decision-making and reduce the training time of new users.
For rheometry measurements, the Discovery HR has a number of temperature systems and temperature chambers, including a relative humidity system which may be useful for measuring hygroscopic hydrogels under different ambient conditions.
The Discovery HR can perform rotational rheology and linear dynamic mechanical analysis in a single instrument under temperature-controlled conditions. The DHR’s excellent sensitivity enables measurements on materials with a range of hardnesses, from steel to more flexible collagens.
To learn more about rheological evaluation of hydrogels read our new rheology of hydrogels application note. Personalized solutions and expert advice are just a call or click away – contact us to learn how TA Instruments rheometers can improve your efficiency and quality control when working with complex biomaterials.
References:
- Baby, D. K. (2020). Chapter 9 – Rheology of hydrogels. In Rheology of Polymer Blends and Nanocomposites. Elsevier Inc. https://doi.org/10.1016/B978-0-12-816957-5.00009-4
- Dong, C., & Lv, Y. (2016). Application of Collagen Scaffold in Tissue Engineering: Recent Advances and New Perspectives. Polymers, 8(42), 1–20. https://doi.org/10.3390/polym8020042
- Meyer, M. (2019). Processing of collagen based biomaterials and the resulting materials properties. BioMedical Engineering OnLine, 18(24), 1–74. https://doi.org/10.1186/s12938-019-0647-0
- Perez-Puyana, V. et al. (2020). Fabrication and Characterization of Hydrogels Based on Gelatinised Collagen with Potential Application in Tissue Engineering. Polymers, 12(5):1146. https://doi.org/10.3390/polym12051146
Other Resources
- Quantifying Polymer Crosslinking Density Using Rheology and DMA
- Characterization of Shape-Memory Polymers by DMA
- Determination of the Linear Viscoelastic Region of A Polymer Using a Strain Sweep on the DMA 2980
- Characterization of an Acrylic/Melamine Copolymer Blend by DSC and DMA
- Characterization of a Polymer Resin/Catalyst System by TGA, DSC, DMA
- Dynamic Mechanical Analyzers
- DMA 850