Lithium-ion Battery Quality and Performance Testing with Isothermal Microcalorimetry
Hang Lau | Morgan Ulrich | Jeremy May | Julienne Regele
April 10, 2023
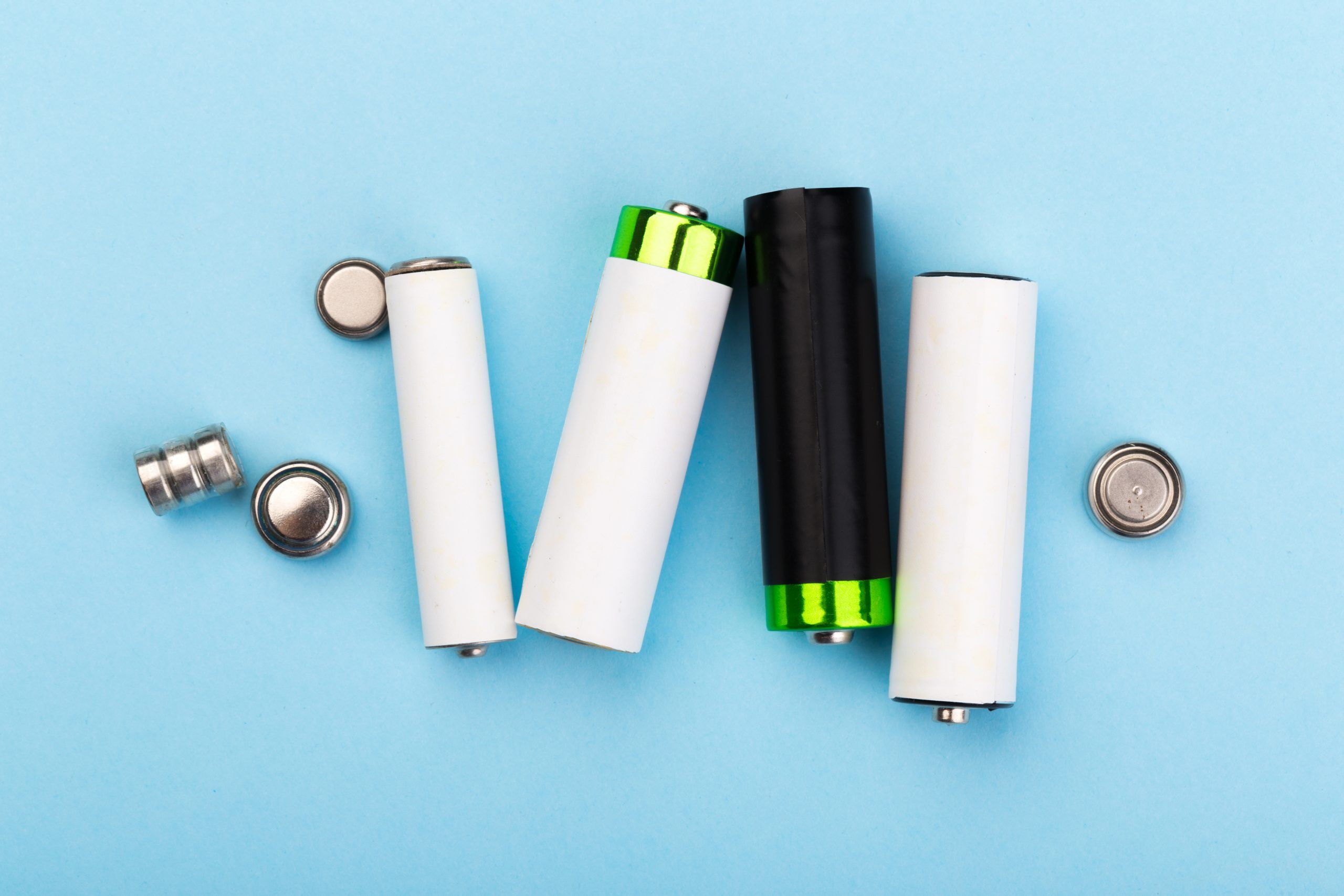
Research breakthroughs powered by parasitic reaction measurements
Over the past decade, battery research, development, and quality control have adopted in-situ and in-operando isothermal microcalorimetry (IMC) as the leading method to evaluate heat flow during lithium-ion battery cycling. While cycling a cell to failure can take many months, emerging diagnostic tests are able to predict long-term behavior in a matter of weeks.
One such emerging diagnostic method is measuring the parasitic heat of a battery during cycling. Krause et al (2012)1 outlined a process to separate parasitic heat events from total heat production, thereby quantifying parasitic reactions. Parasitic reaction data can then be utilized to:
- judge cell quality
- aid in active material formulation
- investigate the impact of additives
- study the formation and growth of the solid electrolyte interphase (SEI)
- contribute to cycle and calendar life prediction models.
The following research examples utilize the TA Instruments TAM (Thermal Activity Monitor) microcalorimeter to evaluate parasitic reactions of batteries with new materials, formulations, and processing methods.
Enhancing New Battery Formulations by Understanding Parasitic Reactions
L. J. Krause et al (2012)1 from 3M and the Jeff Dahn group in Dalhousie University studied the effects of different graphite, as well as the effect of electrode formulation on cell performance. They were early innovators using the TAM III to measure parasitic energy and correlate it to the loss of active lithium, or coulombic efficiency, “confirming the source of the parasitic energy as the heat of reaction occurring between the lithiated electrodes and the electrolyte.” Their method proved effective for studying new material sets and predicting cell lifetime.
Previous works demonstrated that removing ethylene carbonate (EC) from electrolytes in graphite lithium ion pouch cells resulted in increased cycle life and lifetime at high voltage operation. S. L. Glazier et al (2017)2 from Dalhousie University investigated the performance of EC-free electrolytes by measuring parasitic heat flow during high voltage operation on their TAM III microcalorimeter paired with a battery cycler. The team measured the time and voltage dependence of the parasitic reaction to characterize the complex internal reactions in the battery. They found that EC-free electrolytes “yielded higher parasitic heat flow at lower voltages but performed better than EC-containing electrolyte above 4.3 V.” Additionally, the EC-free electrolytes were better able to recover to a lower parasitic heat flow after high voltage exposure. Their work confirms that EC-free electrolytes offer excellent high-performance operation, and further research can help improve their performance at low potentials for a more successful battery electrolyte formulation.
Evaluating New Battery Materials with Heat Flow Measurements in High Voltage
S. L. Glazier et al (2017)3 from Dalhousie University also compared natural graphite and artificial graphite cells by measuring their parasitic heat flows and capacity retention. Their TAM III proved useful for “understanding the voltage and time dependence of parasitic reactions in high voltage lithium ion pouch cells.” They used IMC to study parasitic reactions in a low voltage range to probe the electrolytes’ reaction in the negative electrode, and then they tested in the high voltage range to probe the oxidized positive/negative interaction.
The results demonstrate natural and artificial graphite electrodes with sufficient electrolyte additive loading will produce similar amounts of parasitic heat, with artificial graphite producing the least. Insufficient electrolyte additive loading produced greater parasitic heat flow and significantly worse electrochemical performance in the high voltage range. Long term cycling behavior demonstrated that the natural graphite cells experienced faster rates of capacity fade, relative to artificial graphite. The group proposes that, with insufficient electrolyte loading, the SEI layer is thin and ineffective at withstanding the mechanical expansion of natural graphite particles during lithiation, leading to irreversible expansion and greater rates of capacity fade as new SEI forms on the exposed surfaces.
Developing Baselines for Optimizing High-Ni NMC Cathodes
C. D. Quilty et al (2022)4 also evaluated new lithium-ion battery materials in their study of cells with nickel-rich lithium nickel manganese cobalt oxide (NMC) cathodes. NMC offers high energy density but suffers from high potential capacity fade and therefore its capacity must be carefully limited. Maximizing NMC batteries lifetime and high capacity requires measuring capacity fade mechanisms with a suite of tools, including operando IMC experiments.
C. D. Quilty et al gained a comprehensive understanding of battery degradation through real-time thermal measurements during (de)lithiation with their TAM IV. They noted that IMC helped their research as a “powerful, non-destructive tool to capture the instantaneous heat flow released from a cycling battery with ultra-high precision.” They found that increased rates of capacity fade at higher voltages may be triggered by greater thermal energy waste, or a lower electrochemical efficiency. Their conclusions set the baseline for optimizing future NMC cathodes.
Evaluating Prelithiation impact for New Lithium-Ion Battery Processing Techniques
Prelithiation is a new lithium-ion battery formation method that adds active lithium content prior to battery cell operation. This compensates for lithium loss during the formation cycle, promising high energy density and better cycle performance when done correctly. However, the possible negative side effects of prelithiation are still being studied.
Linghong Zhang et al (2022)5 used a TAM III to assess both the prelithiaton process and the associated parasitic reactions. Prelithiated cells had additional parasitic reactions during the first cycles, but after three cycles, “similar heat signals from parasitic events were observed for the prelithiation cells and the control cells, indicating stabilization and possibility of no long term side effects from prelithiation.”
This study was the first demonstration of isothermal microcalorimetry for evaluating prelithiation and offers promising results about the process. They conclude that “in operando isothermal microcalorimetry is a powerful tool to characterize the prelithiation application for lithium-ion batteries.” Future studies can continue to optimize prelithiation, and it is especially important to monitor the effects of prelithiation additives for safe formation of cells at scale.
The Technology Behind the Research
The five research studies citied above all utilized the TAM Microcalorimeter, a state-of-the-art analysis tool that measures the thermal behavior of samples under controlled temperature conditions. Many of the studies paired the TAM with a potentiostat or battery cycler, enabling them to measure heat flow during battery operation for reliable results.
The new Battery Cycler Microcalorimeter Solution is built with this application in mind, pairing the TAM IV Microcalorimeter with the BioLogic VSP-300 Potentiostat into a single integrated system. Now, researchers and scientists of all levels can measure in-operando battery heat flow with seamless system control and data analytics.
See an example experiment using the new testing solution in this application note: Determination of Parasitic Power in Lithium-ion Batteries using the Battery Cycler Microcalorimeter Solution.
Contact TA Instruments to speak with an expert about your battery testing.
References
- Krause, L. J., Jensen, L. D., Dahn, J. R. (2012). Measurement of Parasitic Reactions in Li Ion Cells by Electrochemical Calorimetry. Journal of The Electrochemical Society, 159 No 7.
https://iopscience.iop.org/article/10.1149/2.021207jes - Glazier, S.L., Petibon, R., Xia, J., Dahn, J.R. (2017). Measuring the Parasitic Heat Flow of Lithium Ion Pouch Cells. Journal of The Electrochemical Society, 164 No 4.
https://iopscience.iop.org/article/10.1149/2.0331704jes - Glazier, S. L., Li, J., Louli, A. J., Allen, J. P., Dahn, J. R. (2017). An Analysis of Artificial and Natural Graphite in Lithium Ion Pouch Cells Using Ultra-High Precision Coulometry, Isothermal Microcalorimetry, Gas Evolution, Long Term Cycling and Pressure Measurements Journal of The Electrochemical Society, 164 No 14. https://iopscience.iop.org/article/10.1149/2.0421714jes
- Quilty, C.D., West, P. J., Li, W., Dunkin, M. R., Wheeler, G. P., Ehrlich, S., Ma, L., Jaye, C., Fischer, D. A., Takeuchi, E. S., Takeuchi, K. J., Bock, D. C., Marschilok, A. C. (2022). Multimodal electrochemistry coupled microcalorimetric and X-ray probing of the capacity fade mechanisms of Nickel rich NMC – progress and outlook. (2022). Physical Chemistry Chemical Physics, 24.
https://pubs.rsc.org/en/content/articlelanding/2022/CP/D1CP05254C - Zhang, L., Chevrier, V. L., Gionet, P., Hung, J., Wu, L., Chen, X., Yu, T., Williams, S., Krause, L. (2022). Isothermal Microcalorimetry Evaluation of In Situ Prelithiation in Lithium-ion Batteries. Journal of The Electrochemical Society, 169.
https://iopscience.iop.org/article/10.1149/1945-7111/aca366
Other Resources
- Application Note – Polymer Melt Rheology Workflow Automation: Auto-Trim for Rheometers
- Application Note – Estimation of Polymer Lifetime by TGA Decomposition Kinetics
- Application Note – Semi-Crystalline Thermoplastic Analysis using an X3 DSC
- Product: Auto-Trim Accessory
- Technique – Rheology
- Application – Polymers